Gastric dilatation volvulus (GDV) is an acute, life-threatening disease with a predisposition in the large breed dog (Bruchim and Kelmer, 2014). The presence of a large gas-filled stomach compromises organ blood supply, resulting in tissue necrosis (Self, 2016). Pressure from a dilated stomach compresses the caudal vena cava resulting in reduced venous return to the heart and a subsequent reduction in cardiac output (Self, 2016). The treatment option for GDV is urgent surgical intervention, but due to the complex aetiology and pathophysiology, cardiovascular stability is crucial to patient outcome (Broome and Walsh, 2003).
Stabilisation
Initial therapy is aimed at stabilisation prior to general anaesthesia, although a prolonged surgical delay is not recommended due to worsening gastric and splenic ischaemia (Broome and Walsh, 2003). Improved cardiovascular and pulmonary function is the objective prior to surgery, but this may not always be possible. Surgical delay may result in arrhythmia and/or myocardial depression and acid–base disturbances potentially being present prior to, during, and following anaesthesia (Broome and Walsh, 2003). Pre-operative gastric decompression is recommended (Figure 1) to improve cardiovascular and respiratory function (Broome and Walsh, 2003), and to stabilise the patient prior to surgical correction.
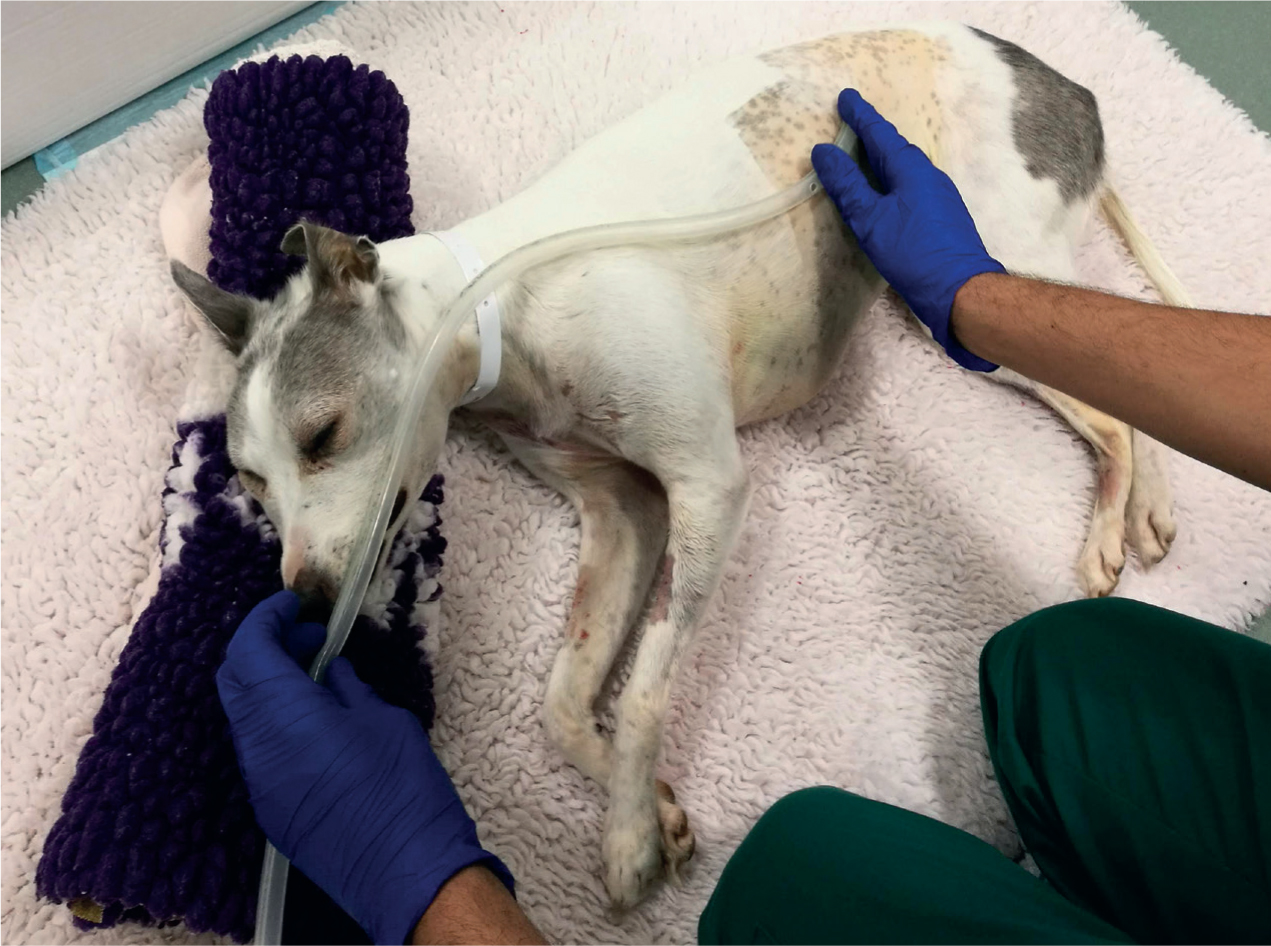
Assessment of the cardiovascular system provides information on oxygen delivery to tissues, but there is no single variable to provide an accurate estimation of efficacy of tissue perfusion. Multiple monitoring methods can be applied according to clinical skill and availability in practice. Subjective methods of assessing cardiovascular function can be easily performed by careful clinical examination including mucous membrane colour, capillary refill time, pulse rate and quality, and core versus peripheral temperature (Pachtinger and Drobatz, 2008). Although not common in general practice, objective measures may include urine output measurement, blood lactate and arterial blood pressure monitoring. Patients presenting with GDV may have clinical signs of shock, including weak pulses, tachycardia, prolonged capillary refill time and pale mucous membranes (Broome and Walsh, 2003). Haak et al (2012) found that 90% of dogs presenting with GDV were tachycardic, and 100% of dogs had abnormal perfusion parameters (mucous membranes, capillary refill time, pulse quality). A number of monitoring techniques are discussed below in order of clinical relevance.
Electrocardiography (ECG)
Cardiac arrhythmias, commonly ventricular in origin (Figure 2), have been reported in up to 40% of dogs with GDV, attributed to myocardial ischaemia (Brockman et al, 1995). Myocardial ischaemia is known to create ectopic foci (abnormal pacemaker sites within the heart) of electrical activity (Sharp and Rozanski, 2014). Although only applicable if bed-side testing is available, circulating cardiac troponins are often elevated, and these biomarkers are sensitive indicators of myocardial ischaemia correlating directly with ECG abnormalities and myocardial injury (Schober et al, 2002). The formation of arrhythmias has also been attributed to increased circulating concentrations of catecholamines and cytokines, and this may explain the cardiovascular instability seen following gastric decompression (Sharp and Rozanski, 2014).
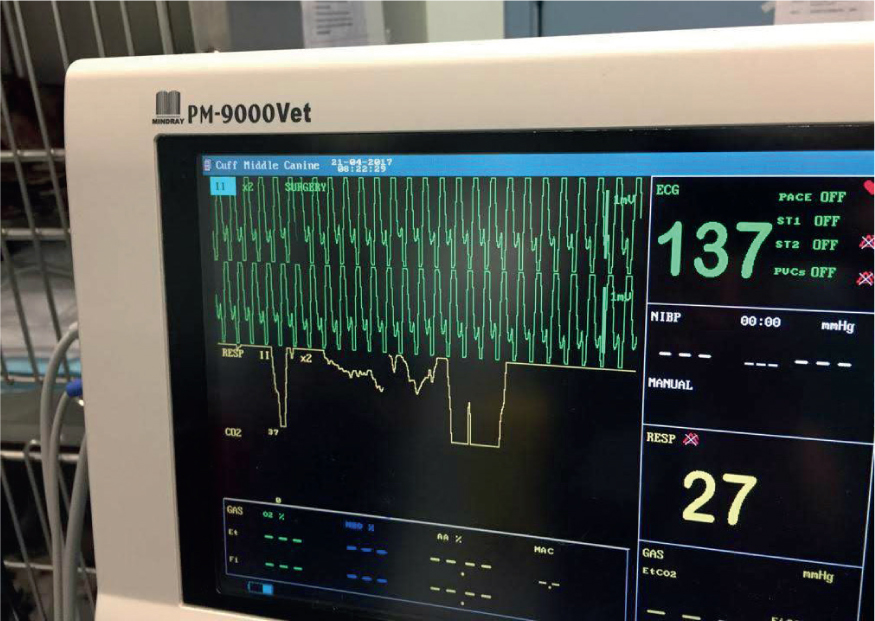
Due to the high incidence of cardiac arrhythmia in GDV, ECG is recommended to identify cardiac arrhythmias and to assess response to treatment. There is discussion regarding whether arrhythmias should be medically managed in the canine GDV patient (Broome and Walsh, 2003), but without ECG, it would not be possible to assess the presence and severity of arrhythmia. Brockman et al (1995) promote the use of antiarrhythmics when ECG abnormalities are associated with poor myocardial function or electrolyte imbalance. This is supported by Volk (2009) who promotes antiarrhythmics if cardiac function and cardiac output are affected. This may be determined by systemic blood pressure monitoring. Bruchim (2012) found that early intervention with a lidocaine bolus followed by constant rate infusion significantly reduced acute kidney injury, cardiac arrhythmia and coagulation disorders. Mortality rates were also reduced.
ECG offers a non-invasive and easy to use method of monitoring cardiac electrical activity. Due to the high incidence of cardiac arrhythmia, even into the postoperative period, ECG monitoring for 24–48 hours following surgery is recommended (Sharp and Rozanski, 2014).
Blood pressure and fluid balance
GDV is characterised by obstructive and relative hypovolaemic and distributive shock which can quickly lead to ischaemia (Bruchim and Kelmer, 2014). Hypovolaemia describes a decreased circulating intravascular fluid volume relative to total vascular space (Cottingham, 2006). This can result from a relative fluid volume deficiency, common in distributive disease states (Cottingham, 2006). Physiologically, the consequence of this is decreased cardiac preload, resulting in reduced cardiac output (CO), poor oxygen delivery and inadequate tissue perfusion (Pachtinger and Drobatz, 2008; Cooper and Cooper, 2012).
Hypotension has been associated with increased mortality in dogs (Haak et al, 2012). Rapid assessment and fluid resuscitation is essential to restore oxygen delivery to hypoxic tissues and correct/prevent hypotension prior to surgery (Haak et al, 2012). Arterial blood pressure is a major tool in assessing haemodynamic status and tissue perfusion (Pachtinger and Drobatz, 2008). Non-direct methods of measuring blood pressure include ultrasonic Doppler and oscillometric measurement (Pachtinger and Drobatz, 2008). Both methods have limitations. Doppler blood pressure will only give information on systolic pressure, while oscillometric blood pressure offers information on systolic, diastolic and mean arterial pressure (MAP). MAP is considered a good indicator of driving pressure for tissue perfusion, but it is important to remember that a normal MAP will not rule out poor tissue perfusion (Cooper and Cooper, 2012). This is because MAP is not an isolated value and is dependent on cardiac output and systemic vascular resistance (SVR) (Pachtinger and Drobatz, 2008). Compensatory responses can increase cardiac output and SVR to normalise MAP despite a reduced perfusion state (Pachtinger and Drobatz, 2008).
Direct arterial blood pressure (DABP) measurement requires the placement of an arterial catheter attached to a pressure transducer. This allows a continuous measurement value and pressure waveform (Clapham, 2011). Arterial blood pressure measurement is considered the ‘gold standard’ method of direct blood pressure measurement and gives the most accurate results (Haskins, 2011). DABP measurement is indicated for critical patients and high risk anaesthetic cases due to its accuracy and continuous results (Waddell and Brown, 2015). However, DABP measurement requires specialist equipment and clinical skills which may not be available. This technique does have associated risks including haemorrhage, haematoma formation, infection, thrombosis and possible tissue necrosis (Wagner and Brod-belt, 1997; Mazzaferro and Wagner, 2001).
Cardiac output
Cardiac output is defined as the amount of blood pumped into the aorta each minute (Hall, 2016a). When assessing circulation and adequate tissue perfusion, cardiac output is the most important consideration because it quantifies the amount of blood flow to all body tissues (Hall, 2016a). The cardiac reserve describes the maximum percentage of cardiac output increase above normal (Hall, 2016b). In the healthy human, this can be up to 400% (Hall, 2016b). There is no known cardiac reserve data for veterinary species, but it is known that in humans with compromised cardiac function, the cardiac reserve is greatly decreased, and absent in some cases (Hall, 2016b). It is, however, important to consider that any physiological process that prevents normal cardiac function will reduce cardiac reserve (Hall, 2016b). Cardiac output is an important indicator of circulatory adequacy (Haskins, 2011), but because cardiac output is a flow parameter and not a pressure parameter, it is challenging to measure.
Assessing the efficacy of the cardiovascular system and taking steps to improve function is an essential element of pre, peri and postoperative management (Kong and Vetrugno, 2012). Realistically, noninvasive measurements of cardiovascular parameters (blood pressure measurement and pulse quality) offer limited information on cardiovascular function. It has been recognised that trying to interpret cardiac output from these parameters is inaccurate and of little use for therapeutic intervention (Kong and Vetrugno, 2012).
Kong and Vetrugno (2012) discussed the variable methods of monitoring cardiac output including lithium dilution and pulse contour analysis. There are a variety of devices available ranging in technique and invasiveness. Unfortunately, many of the devices discussed are currently only used in human anaesthesia (Boag and Hughes, 2005). Boag and Hughes (2005) discussed the ‘gold standard’ approach to monitoring tissue perfusion which involves placement of a pulmonary arterial catheter, inserted into a central vein (femoral or jugular) to allow measurement of cardiac output, pulmonary vascular resistance, right atrial pressure, oxygen saturation and delivery. Realistically, these advanced techniques are not available in most veterinary practices, and so different techniques may be required (Boag and Hughes, 2005).
Although not common at present, thermodilution is the technique of choice for cardiac output monitoring in dogs (Kong and Vetrugno, 2012). Thermodilution involves the placement of a pulmonary arterial catheter and subsequent administration of cold saline solution via a port into the right atrium. The temperature difference is measured, and a curve of results is generated. Although reliable, this technique is not available in all settings, likely due to cost, clinical skill and the invasive nature of the procedure. Mantovani et al (2017) compared thermodilution technique with transoesophageal echocardiography (TOE) to monitor perioperative cardiac output in dogs. The results of the study indicated TOE to be a good non-invasive alternative to thermodilution. This is promising research, which may help to promote the benefits of cardiac output measurements in veterinary species. TOE is a routine procedure in human medicine, and increasing in popularity for cardiovascular procedures in referral veterinary centres.
Capnography and pulse oximetry
Capnography offers a continuous and non-invasive measurement of respiratory carbon dioxide (CO2), providing information on ventilatory function and perfusion status (Swenson et al, 2008). Ornato et al (1990) found that the relationship between CO2 and cardiac output is logarithmic, and this may mean that end tidal CO2 can offer valuable information on cardiac output during low flow states, but only during physiologically normal ventilation.
Pulse oximetry is also a non-invasive method and allows an estimate of arterial haemoglobin saturated with oxygen (Thawley and Waddell, 2013). The limitations of pulse oximetry are apparent when compared with blood gas analysis, but it is still a useful tool for anaesthetised patients (Thawley and Waddell, 2013). The ease of use and straightforward results make pulse oximetry a popular addition in most settings, however, the result can be unreliable in the face of low perfusion states, skin pigmentation, concurrent use of electrosurgical devices and electrical interference (Brockman et al, 1995).
Blood gas analysis
Acid–base disturbances can be seen in dogs with GDV (Wingfield et al, 1975), although Volk (2009) found that such disturbances were not consistent. Experimental studies in induced gastric dilatation and GDV have shown metabolic acidosis to be a common finding (Wingfield et al, 1975). This can be attributed to the production of lactic acid from poorly perfused tissues (Merkley et al, 1976). Wingfield et al (1975) demonstrated that because of the occurrence of opposing primary disturbances, blood pH can be normal. Wingfield et al (1975) found that during naturally occurring GDV, acid–base was balanced with metabolic alkalosis, attributed to damaged gastric mucosa and an outpour of hydrogen ions within the gastric lumen. Hypokalaemia can be present and attributed to one of several factors including intravenous fluid resuscitation with low potassium content solutions, sequestration of potassium in the stomach or losses through vomiting/gastric lavage (Sharp and Rozanski, 2014). Hypokalaemia can worsen cardiac arrhythmias and result in ileus, so it is beneficial to measure potassium in the postoperative period, and supplement as required (Broome and Walsh, 2003). Although arterial blood gas analysis can offer an accurate method of assessing oxygenation and ventilation, it only offers a snapshot of time, and so repeated sampling is required (Thawley and Waddell, 2013).
The assessment of acid–base and electrolyte parameters can provide crucial information for the treatment plan during anaesthesia and hospitalisation. Although not specific to myocardial injury, an increased plasma lactate concentration can be seen with decreased tissue oxygen delivery (de Papp et al, 1999) and hypoperfusion of tissues leads to anaerobic metabolism (Beer et al, 2013). De Papp et al (1999) demonstrated a link between high plasma lactate concentrations and increased gastric necrosis, making it a useful parameter for assessment.
Postoperative considerations
The postoperative period also requires careful observation and should continue to be focused on maintaining tissue perfusion (Bruchim and Kelmer, 2014). Cardiac arrhythmia can occur into the postoperative period, so postoperative ECG may be justified. Patients with GDV can develop systemic inflammatory response syndrome, which can cause multiple organ dysfunction syndrome (Osterbur et al, 2014). Organ dysfunction has been documented in the cardiovascular, respiratory, gastrointestinal, coagulation and renal systems (Sharp and Rozanski, 2014). Cardiovascular effects of GDV include shock, arrhythmia and myocardial deterioration (Sharp and Rozanski, 2014). The primary systemic side effects contributing to early morbidity and mortality in dogs with GDV are attributed to cardiovascular compromise (Sharp and Rozanski, 2014).
Conclusion
Haemodynamic and cardiac output assessment is a crucial element of critical case management, but realistically, some of the gold standard methods discussed are not available to all anaesthetists and nurses. This promotes the need to work with non-invasive devices available and interpret the information to gain an overview of haemodynamic status, at the advantage of the patient and veterinary staff (Burrows and Ignaszewski, 1990).
KEY POINTS
- Gastric dilatation volvulus (GDV) is an acute and life threatening disease, requiring immediate surgical intervention.
- Pre-operative gastric decompression can improve cardiovascular and pulmonary function prior to surgical intervention.
- Assessment of the cardiovascular system can provide information on the efficacy of tissue perfusion.
- The veterinary nurse plays a crucial role in managing the GDV patient, from pre operative stabilisation to postoperative care.