Some poisons can result in significant cardiac and/or cardiovascular complications. These include drugs for cardiac and cardiovascular conditions, drugs used in the management of other diseases, over-the-counter medicines and supplements, foods and natural toxins such as plants (Box 1). Some of the more common substances and those requiring complex management are discussed here. Terms are explained in Table 1.
Box 1.Potential toxicological causes of cardiac or cardiovascular effects in companion animals
- Many drugs for treating arrhythmias or hypertension
- 5-hydroxytryptophan (5-HTP)
- 5-fluorouracil
- Sympathomimetics (phenylephrine, phenylpropanolamine, ephedrine, pseudoephedrine)
- Tricyclic antidepressants
- Baclofen and other muscle relaxants
- Amphetamines
- Vitamin D compounds (as a result of calcification of cardiac tissue)
- Plants containing cardiac glycosides such as foxglove (Digitalis species), oleander (Nerium oleander), Lily-of-the-valley (Convallaria majalis)
- Yew (Taxus baccata)
- Alphachloralose
- Adder bite
- Bufo marinus (cane toad)
- Insulin overdose (as a result of hypokalaemia)
Table 1. Definitions of terms used
Term | Meaning or effect |
---|---|
Agonist | In pharmacology, a drug that binds to a receptor and activates the receptor to produce a biological response |
Antagonist | In pharmacology, a drug that blocks the effect of an agonist |
Arrhythmia | A disorder of the heart that affects the rate or rhythm at which the heart beats |
Cardiac output | The amount of blood the heart pumps through the circulatory system in a minute |
Cardioselectivity | Refers to drugs, mainly beta-blockers, that have multiple pharmacological effects but act mainly on the cardiovascular system |
Chronotropic effect | Decrease in heart rate |
Extrasystoles | Additional heartbeats that occur outside the normal heart rhythm |
Hypoperfusion | Reduced blood flow |
Idioventricular rhythms | A slow regular ventricular rhythm, typically with a rate of less than 50 beats per minute |
Junctional rhythm | A junctional rhythm occurs when the electrical activation of the heart originates near or within the atrioventricular node, rather than from the sinoatrial node (a specialised heart structure that initiates the electrical impulses to stimulate contraction) |
Myocardial contractility | The ability of the heart muscle to contract |
Negative inotropic effect | Reduction in blood pressure |
Paroxysmal tachycardia | A type of irregular heartbeat |
Paroxysmal rhythms | Paroxysmal means that the episode of arrhythmia begins and ends abruptly |
Partial agonist | Drugs that bind to and activate a given receptor but have only partial efficacy and produce submaximal response at the receptor compared to a full agonist |
QT prolongation | An electrical disturbance that can be seen on an electrocardiogram, where the heart muscle takes longer than normal to recharge between beats |
Selectivity | Selectivity refers to a drug's ability to preferentially produce a particular effect and is related to the structural specificity of drug binding to receptors |
Torsade des pointes | A specific type of abnormal heart rhythm that is a polymorphic ventricular tachycardia (a fast, abnormal heart rate starting in the ventricles) that shows distinct characteristics on the electrocardiogram. It usually terminates spontaneously but frequently recurs and may degenerate into ventricular fibrillation |
Ventricular arrhythmias | Abnormal heartbeats that originate from the lower heart chambers (the ventricles) |
Ventricular fibrillation | An abnormal heart rhythm caused by disorganised electrical activity in which the ventricles of the heart quiver instead of pump. It is a serious cardiac disturbance that can be fatal |
Ventricular premature contractions | Where the heartbeat is initiated by Purkinje fibres in the ventricles (two large chambers toward the bottom of the heart) rather than by the sinoatrial node (a specialised heart structure that initiates the electrical impulses to stimulate contraction, which is located in the right atrium (one of the upper chambers) of the heart) |
Animals with pre-existing cardiac disease are likely to be more at risk of adverse effects after exposure to a cardio-toxin. In addition, animals with a mixed cardiotoxic drug overdose (e.g. a calcium-channel blocker and a beta-blocker) are also more at risk. This is more likely to occur if a dog raids a medicine supply (e.g. a repeat prescription delivered through the letterbox, contents of a dosette box) rather than simply ingesting a dropped tablet.
Effects on specific receptors
Salbutamol
Salbutamol (albuterol) is a selective beta-2(β2)-adrenoceptor agonist with effects on both smooth and skeletal muscle. It is widely used in human medicine as a bronchodilator for respiratory conditions. Dogs are commonly exposed when they bite and pierce an inhaler containing salbutamol (Figure 1) since these devices are usually readily accessible.
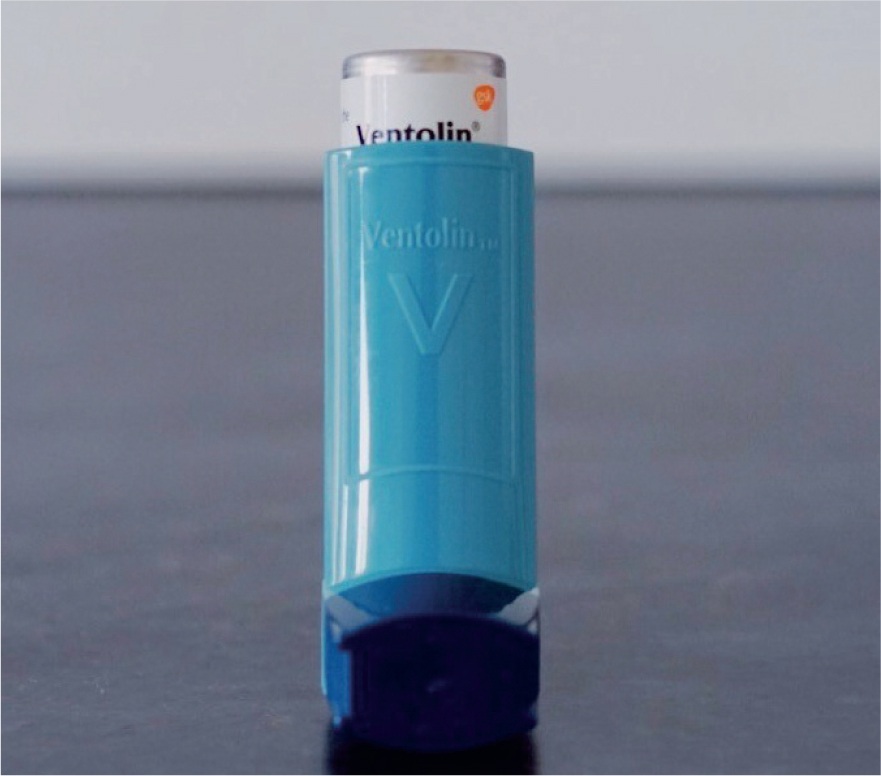
Mechanisms of toxicity
There are two types of β-receptors (β1 and β2) and they are distributed in different tissues. The clinical effects of salbutamol toxicosis are a direct result of the β2-agonist activity of the drug and at therapeutic concentrations, salbutamol is selective for β2 adrenergic receptors, primarily causing relaxation of bronchial, vascular and uterine smooth muscle. At higher doses, the β2 selectivity of salbutamol may be reduced, with activation of β1 adrenergic receptors, resulting in cardiovascular stimulation (Stiles and Plumb, 1993; Libretto, 1994). Increases in heart rate seen with salbutamol exposure include a reflex response to systemic hypotension resulting from salbutamol-induced peripheral and coronary vasodilation (Nayler and McInnes, 1972; Petruska et al, 1997).
Salbutamol can also cause hypokalaemia, possibly as a result of cyclic adenosine monophosphate-mediated stimulation of the Na+/K+ adenosine triphosphate (ATP) pump (Whyte et al, 1987; Vite and Gfeller, 1994). The decrease in serum potassium levels is transient and total body potassium does not decrease (Vite and Gfeller, 1994). The low serum potassium concentration can result in muscle weakness and flaccid paralysis (Vite and Gfeller, 1994). Salbutamol-induced hypokalaemia can also cause arrhythmias and reduced urinary acid secretion resulting in metabolic acidosis (McCown et al, 2008).
Clinical effects
The onset of effects after exposure to salbutamol can be rapid, usually 30 minutes to 4 hours in most cases.
The most common features in cases of salbutamol toxicosis in dogs are tachycardia, tachypnoea, vomiting, lethargy, panting, hypokalaemia and tremor (Crouchley and Bates, 2016). Other reported effects include polydipsia, collapse, weakness, ataxia, excitability, hyperaesthesia, peripheral vasodilation, twitching, depression, conjunctival hyperaemia, vocalisation, hyperpnoea or hyperventilation and hypersalivation (Crouchley and Bates, 2016). Salbutamol can cause a transient hyperglycaemia followed by an increase in serum insulin levels.
Hypokalaemia can result in muscle weakness and flaccid paralysis (Vite and Gfeller, 1994; McCown et al, 2008) and arrhythmias (Romito et al, 2013) (Box 2).
Box 2.Case reportsSalbutamolA 4-year-old Labrador Retriever guide dog was found panting and distressed, with a punctured can of salbutamol. On presentation (up to 12 hours after exposure), the dog had a tachyarrhythmia with femoral pulse deficits. The dog was treated with intravenous (IV) lactated Ringer's solution and referred to the emergency centre where it was found to have significant tachycardia (180 bpm) with pulse deficits. An electrocardiogram showed paroxysmal ventricular tachycardia and multiple premature ventricular complexes (PVCs), and the dog was hypokalaemic (2.47 mmol/litre, range 3.6–5.8 mmol/litre). The dog was treated with lactated Ringer's solution supplemented with potassium chloride, oxygen and propranolol. The tachycardia and PVCs reduced, but the ventricular tachycardia was persistent with runs of up to 30–40 seconds. Propranolol was changed to lidocaine, which reduced the frequency of the arrhythmia. After 7 hours, the potassium had normalised and the potassium supplementation was reduced to maintenance rate. Lidocaine was continued overnight, then the dose was reduced as the dog had improved, however this caused the ventricular tachycardia to return and lidocaine was therefore increased again. After 24 hours (2 days after presentation) the lidocaine was once again reduced, then reduced further over the next 12 hours with only occasional PVCs. Lidocaine was then stopped (36 hours after presentation) and the dog was observed for a further 24 hours, where only occasional PVCs were seen. The dog was then discharged (3.5 days after initial presentation) and was clinically normal 7 days later (Mitten et al, 1999).Calcium-channel blockerA 4-year-old Pomeranian presented 2.5 hours after ingestion of a diltiazem extended-release capsule (79 mg/kg) and was given an emetic. Clinical signs of progressive hypotension and severe bradycardia with atrial standstill (by 6 hours post-ingestion) were observed, which persisted despite treatment with IV fluids, atropine, calcium, glucagon, and dopamine. She was given high-dose insulin therapy (at 10 hours) and lipid emulsion (at 6 hours for 20 hours). Within 1 hour of initiating high-dose insulin therapy, the clinical signs improved, and with continued treatment, she remained normotensive. She was discharged 45 hours after admission (Maton et al, 2013).CaffeineA 29.4 kg husky ingested 30–60 capsules of raspberry ketones and caffeine. From 30 minutes she developed vomiting and rapidly progressed to panting, ataxia, twitching, cyanosis, seizures, cardiac arrest and died 1.5 hours post-ingestion. Both diazepam and adrenaline were ineffective (MacCallum et al, 2017).
Some metered-dose inhalers contain compressed gas and this can occasionally cause significant oral thermal injury in dogs. The onset can be delayed by 24 hours and oedema, ulceration and erythema of the soft palate, extensive pharyngeal and laryngeal oedema, resulting in increasing respiratory distress, have been reported (Mackenzie et al, 2012). This is not common but mild signs of facial, oral or ocular inflammation may be seen.
Recovery from salbutamol toxicosis usually occurs within 8–24 hours, but occasionally can be longer. A case described in the literature reported cardiac effects (persistent extrasystoles, phases of idioventricular rhythm of slow heart rate and paroxysmal ventricular tachycardia) that lasted up to 2 months in a dog after acute salbutamol exposure, despite correction of hypokalaemia within 19 hours after exposure (Matos et al, 2012).
Treatment
Treatment of salbutamol overdose is aimed at providing supportive care based on clinical signs and controlling tachycardia.
Gut decontamination is usually not required for salbutamol exposure since many cases result from puncturing an asthma inhaler. Activated charcoal can be considered if an oral formulation has been ingested within an hour, provided the animal is asymptomatic.
Monitoring of animals with salbutamol over-exposure should include the heart rate, blood pressure and electrocardiogram (Stiles and Plumb, 1993). If clinical effects develop, then the electrolytes, particularly the potassium concentration, should be checked and corrected. Note that initial correction of a low potassium concentration does not involve potassium supplementation. Salbutamol causes a transient decrease in serum potassium and this does not reflect a decrease in total body potassium. Therefore, a beta-blocker is a more specific treatment for salbutamol-induced hypokalaemia than potassium supplementation (Vite and Gfeller, 1994). Propranolol is preferred over atenolol since it is not selective for β1-receptors but will act on both β1 and β2, and will help control systemic signs, hypokalaemia and cardiac effects. If the potassium concentration falls below 2.5 mmol/litre, however, then it is appropriate to correct with intravenous (IV) potassium supplementation. Also, caution is advised when administering IV fluids without checking potassium concentrations, since IV fluid therapy may further dilute potassium and exacerbate signs of hypokalaemia (Vite and Gfeller, 1994).
If tachycardia is prolonged or severe, or if there are signs of reduced cardiac output (pale mucous membranes, weak pulses, cold extremities; Stiles and Plumb, 1993) then a beta-blocker, preferably propranolol, is recommended. If arrhythmias occur despite propranolol administration, lidocaine can be given (Mitten et al, 1999). If lidocaine is used instead of propranolol, however, potassium concentrations should be monitored closely as lidocaine will not correct hypokalaemia (Mitten et al, 1999).
In dogs with severe or persistent tachycardia consider checking troponin concentrations because myocardial damage can lead to cardiac sequelae in salbutamol overdose. If myocardial injury is suspected or confirmed and there are persistent arrhythmias, an antiarrhythmic drug may be required, e.g. propranolol.
Intravenous fluids can be given for shock or collapse, if appropriate, with monitoring of electrolytes.
Oral thermal injury may be delayed in onset, therefore once discharged the owner should be advised to observe for any signs associated with oral injury (inappetence, anorexia, adipsia, dysphagia, respiratory distress) and to return if they occur (Mackenzie et al, 2012). Management is supportive.
Beta-blockers
Beta-receptor antagonists (beta-blockers) such as atenolol, propranolol and bisoprolol, are used in veterinary and human medicine in the management of hypertension, hyperthyroidism, glaucoma, arrhythmias and cardiomyopathy.
Mechanisms of toxicity
Beta-blockers exert their effects by antagonising the effects of catecholamines at beta-adrenergic receptors. β1- receptors in the heart control heart rate and contractility, regulate heart rate and blood pressure. Beta-blockade of these receptors, therefore, will cause negative inotropic (reduce blood pressure) and chronotropic effects (decrease heart rate).
As noted earlier, β2-receptors are present in smooth muscle including bronchial and vascular smooth muscle. β2-receptors in the liver control glycogenolysis and gluconeogenesis. Beta-blockade of β2-receptors therefore results in an increase in airway resistance, reduced breakdown of muscle glycogen and inhibition of catecholamine-dependent lipolysis.
Beta-blockers mediate potassium uptake into skeletal muscle, liver cells and adipose tissue. β2 agonists (such as salbutamol) stimulate Na+/K+ ATP, which prompts potassium uptake into skeletal muscles. Increased insulin secretion by beta-blockers can further increase cellular potassium uptake. Beta-blockade interferes with these processes (hence their usefulness in salbutamol overdose, as noted above) and may produce hyperkalaemia in overdose (Anderson, 2008).
Variations in the pharmacological properties (cardioselectivity, partial agonist, membrane stabilising activity and lipid solubility) influence the therapeutic actions and side effects of the different beta-blocker drugs.
Clinical effects
Clinical signs of beta-blocker poisoning can occur within 6 hours of ingestion (8 hours for sotalol) or up to 12 hours for modified release tablets that have been swallowed whole.
Hypotension and bradycardia are the most common effects seen in overdose, but beta-blockers with partial agonist activity (pindolol, celiprolol) may lead to tachycardia. Other effects include central nervous system depression, respiratory depression, convulsions, pulmonary oedema, arrhythmias (especially atrioventricular block) and asystole. These effects are uncommon in beta-blocker poisoning in dogs.
Hypoglycaemia and hyperkalaemia may be seen but are uncommon in beta-blocker poisoning. Azotaemia and elevated liver enzymes may occur following prolonged hypotension and decreased tissue perfusion, and acidosis can result from poor cardiac perfusion.
The effects of sotalol in overdose are different as it is a beta-blocker with class III anti-arrhythmic activity. Cases are rarely reported in dogs but in human cases sudden deterioration can occur. Signs reported in humans include hyperkalaemia and hypoglycaemia, drowsiness, metabolic acidosis, coma, convulsions, respiratory depression, bradycardia with or without hypotension, cardiovascular collapse and arrhythmias.
Treatment
Treatment of beta-blocker poisoning is aimed at gut decontamination, providing supportive care based on clinical signs and supporting cardiovascular function.
A therapeutic dose, particularly in a drug-naive animal, will cause beta-blockade and exertion could potentially cause sudden and transient weakness and collapse. It is therefore advised to warn the owner of this potential risk and to suggest reduced exercise/cage rest for a few hours following ingestion of a beta-blocker.
If ingestion was recent (within 1–2 hours) an emetic can be given followed by activated charcoal (1–3 g/kg). Repeated doses of activated charcoal may be of benefit if a sustained released preparation has been ingested (1 g/kg every 4–6 hours for 2–4 doses).
Monitoring should include blood pressure, heart rate and electrocardiogram (to assess presence of conduction abnormalities), blood glucose and potassium concentrations. It is also important to monitor for fluid overload with frequent assessment of respiratory effort and rate and lung sounds. In addition, animals with sotalol overdose and significant signs will require monitoring of blood gases.
Hypotension should initially be treated with intravenous fluids but this may be ineffective in animals with bradycardia. Atropine can be used in dogs with bradycardia (<50 bpm), but it may be resistant to atropine therapy (Peterson, 2016). In animals unresponsive to intravenous fluids and atropine, the use of insulin/dextrose therapy and/or lipid emulsion should be considered (Table 2). High dose insulin therapy is commonly used in humans with beta-blocker poisoning who are unresponsive to other therapies (Karthik et al, 2015; Cole et al, 2018; Seegobin et al, 2018; Rotella et al, 2020) and there is good evidence in animal models for its efficacy in beta-blocker toxicosis (Kerns et al, 1997; Harvey et al, 2011; Cole et al, 2013). It is unlikely to be required in the majority of cases in dogs since severe cases are uncommon.
Table 2. Drug dosages for severe beta-blocker or calcium-channel blocker poisoning
Drug | Dosage |
---|---|
Atropine | 0.02–0.04 mg/kg intravenously (IV) |
Calcium salts | Calcium-channel blocker poisoning
|
Calcium gluconate50–150 mg calcium gluconate/kg equivalent to 0.5–1.5 ml/kg of a 10% solution IV over 20–30 minutes (equivalent to 4.5–14 mg calcium/kg or 0.11–0.34 mmol calcium/kg)A constant rate infusion of up to 0.05–0.15 ml/kg/hour can be given after the bolus and titrated to effect | |
Calcium borogluconate50–150 mg calcium borogluconate/kg equivalent to 0.5–1.5 ml/kg of a 10% solution IV over 20–30 minutes (equivalent to 3.8–11.4 mg calcium/kg or 0.11–0.34 mmol calcium/kg) | |
Insulin/dextrose (Peterson, 2016) |
|
Lipid emulsion | The typical dosage (regimen 1)1.5 ml/kg of a 20% lipid emulsion is given intravenously as a bolus dose. This is followed by an infusion (0.25 ml/kg/minute over 30–60 minutes). The infusion can be repeated once or twice, with additional bolus doses, if required. If no response, lipid can be discontinued. If there is a response, the bolus and/or the infusion can be repeated when signs recur, provided the blood is not lipaemicAn alternative dose for ingested substances (regimen 2)2.25 ml/kg initial loading dose of a 20% lipid emulsion followed by 0.025 ml/kg/minute constant rate infusion for up to 6.5 hours (Fettiplace et al, 2015) |
Glucagon (Peterson, 2016) |
|
AdrenalineNoradrenalineDobutamineDopamine | Beta-blocker poisoning: not recommended for the treatment of beta-blocker-induced hypotensionLess effective than insulin/dextrose or lipid emulsion and may be harmful (Peterson, 2016) |
NoradrenalineDobutamineDopamine | Calcium channel blocker poisoningOnly to be used if blood pressure fails to response to calcium, lipid or insulin therapy (Peterson, 2016)
|
Magnesium | For sotalol-induced torsades des pointes0.15–0.3 mEq/kg (0.075–0.15 mmol/kg) IV administered over 5–15 minutes |
Intravenous lipid emulsion therapy (Table 2) has been used successfully in humans with beta-blocker overdose (Barton et al, 2015; Walter et al, 2018) and experimental animals (Macala et al, 2018; Katzung et al, 2019). In experimental studies, lipid emulsion appeared to be useful even in poisoning with beta-blockers that were not lipophilic, such as atenolol (Cave and Harvey, 2009).
Other therapies include diazepam for convulsions and airway protection, and ventilatory support for animals with seizures, respiratory depression or decreased mental status if required.
Management of sotalol toxicosis is different and drug therapy should be avoided if possible because of the risk of worsening features of overdose. Use of class Ia (quinine, quinidine, procainamide, disopyramide) and class III (amiodarone) anti-arrhythmic drugs is contraindicated since they may precipitate further changes in the QT interval of the electrocardiogram. Lidocaine is also contraindicated as it further reduces the blood pressure. Magnesium can be given for torsade des pointes (Table 2).
Calcium-channel blockers
Examples of calcium-channel blockers are amlodipine, diltiazem and verapamil. Some calcium-channel blockers are used in veterinary medicine for hypertension and cardiac disease. Severe cases of poisoning in dogs have been reported (Syring et al, 2008; Maton et al, 2013; Becker and Young, 2017) but are not common.
Mechanism of toxicity
Calcium-channel blockers act by inhibiting movement of calcium ions from extracellular sites through the membrane-based calcium channels. All these drugs decrease arterial vascular resistance and thereby lower the blood pressure. Diltiazem, and particularly verapamil, also decrease resting heart rate and myocardial contractility (the ability of the heart muscle to contract).
Clinical signs
Signs of calcium-channel blocker poisoning usually occur within 6 hours of ingestion, even with sustained release preparations. Recovery is usually within 6–24 hours, but can be longer in animals with significant signs.
Verapamil and diltiazem can cause bradycardia and/or bradyarrhythmias (all degrees of heart block, QT prolongation or junctional rhythms) and hypotension in overdose. Sinus tachycardia may also occur (likely as a result of carotid sinus reflex stimulation). The other calcium-channel blocker drugs cause hypotension (because of vasodilation) and reflex tachycardia but bradycardia may occur after ingestion of a large dose (as a result of depression of nodal conduction).
In addition, all calcium-channel blockers may cause vomiting and diarrhoea, hypothermia, weakness and collapse in overdose. Hyperglycaemia (resulting from an inhibition of insulin release), increased blood lactate levels (because of impaired cardiac output and glucose entry into cells), hypokalaemia and metabolic acidosis (because of tissue hypoperfusion) may also occur. Depression, coma and convulsions may be seen because of decreased cardiac output, hypotension and reduced cerebral perfusion (Holder, 2000). In severe cases there may also be respiratory distress and non-cardiogenic pulmonary oedema.
Treatment
Treatment of calcium-channel blocker poisoning is aimed at gut decontamination, providing supportive care based on clinical signs and augmenting myocardial function (Hayes and Knight, 2012).
Induction of emesis is contraindicated in any animal with bradycardia, hypotension or central nervous system depression (Costello and Syring, 2008). Otherwise, and depending on the condition of the animal, an emetic may be given if within 1–2 hours of ingestion followed by activated charcoal. Further doses of activated charcoal may be of benefit if a sustained release preparation has been ingested or if the animal is symptomatic (as a result of possible enterohepatic recirculation) (1–3 g/kg every 4–6 hours for 2–4 doses).
Monitoring should include heart rate, rhythm and blood pressure, and blood glucose and lactate concentrations in symptomatic animals. Renal parameters should be checked daily in symptomatic dogs (Peterson, 2016). Animals with calcium-channel blocker poisoning will not have an adequate cardiac output; therefore, it is important to monitor for fluid overload (Peterson, 2016) by monitoring bodyweight, although in animals with significant signs, placement of a central line to monitor central venous pressure is recommended (Costello and Syring, 2008).
In animals with significant signs, the respiratory status (respiratory rate, effort, auscultation and blood gas analysis) should be monitored because of the risk of hypoxaemia as a result of fluid overload or non-cardiogenic pulmonary oedema. Symptomatic animals should be monitored for 12–24 hours or longer (Hayes and Knight, 2012).
Intravenous fluids should be given to support cardiovascular function in calcium-channel blocker overdose (Hayes and Knight, 2012), but should be done with care to prevent fluid overload (particularly in patients with pre-existing cardiac disease) and because of the risk of non-cardiogenic pulmonary oedema. For persistent hypotension and/or bradycardia, calcium salts are recommended. Calcium salts may be of benefit for dogs that are haemodynamically unstable and is the first line treatment in symptomatic animals (Table 2). Glucagon has been used in humans with calcium-channel blocker poisoning unresponsive to calcium salts and could be considered in dogs (however, large doses may be required and may not be available). In animals unresponsive to these therapies, insulin/dextrose therapy and/or lipid emulsion should be considered (Table 2). High dose insulin therapy is commonly used in humans with calcium-channel blocker poisoning, and there is good evidence in animal models for its efficacy in calcium-channel blocker toxicity (Kline et al, 1993, 1995). In severe cases that are unresponsive to supportive therapy, lipid emulsion therapy should be considered. It has been used successfully in dogs with calcium-channel blocker overdose (Maton et al, 2013; Becker and Young, 2017) (Box 2) and experimental studies (Tebbutt et al, 2006; Bania et al, 2007).
Other treatments include diazepam or a barbiturate for convulsions, and oxygen is recommended for animals with pulmonary oedema. If hyperglycaemia persists for more than a few hours, it should be controlled with insulin; insulin also has positive inotropic effects (it increases the strength of muscular contractions) (Holder, 2000). Potassium should be supplemented if it falls below 2.5 mmol/litre (Hayes and Knight, 2012). Severe heart block may require placement of a temporary cardiac pacemaker (Hayes and Knight, 2012); temporary transvenous pacing was required in a dog with severe diltiazem poisoning, resulting in persistent bradycardia, hypotension and AV block (Syring et al, 2008).
Myocardial stimulation
Caffeine and theobromine
Caffeine and theobromine are both methylxanthines. Caffeine is found in coffee, tea, many soft drinks, as well as in several over-the-counter stimulant tablets, human cold medicines, analgesics and weight loss products (Figure 2). Theobromine is the toxic component in chocolate (Figure 3). Both compounds are metabolised to other methylxanthines.
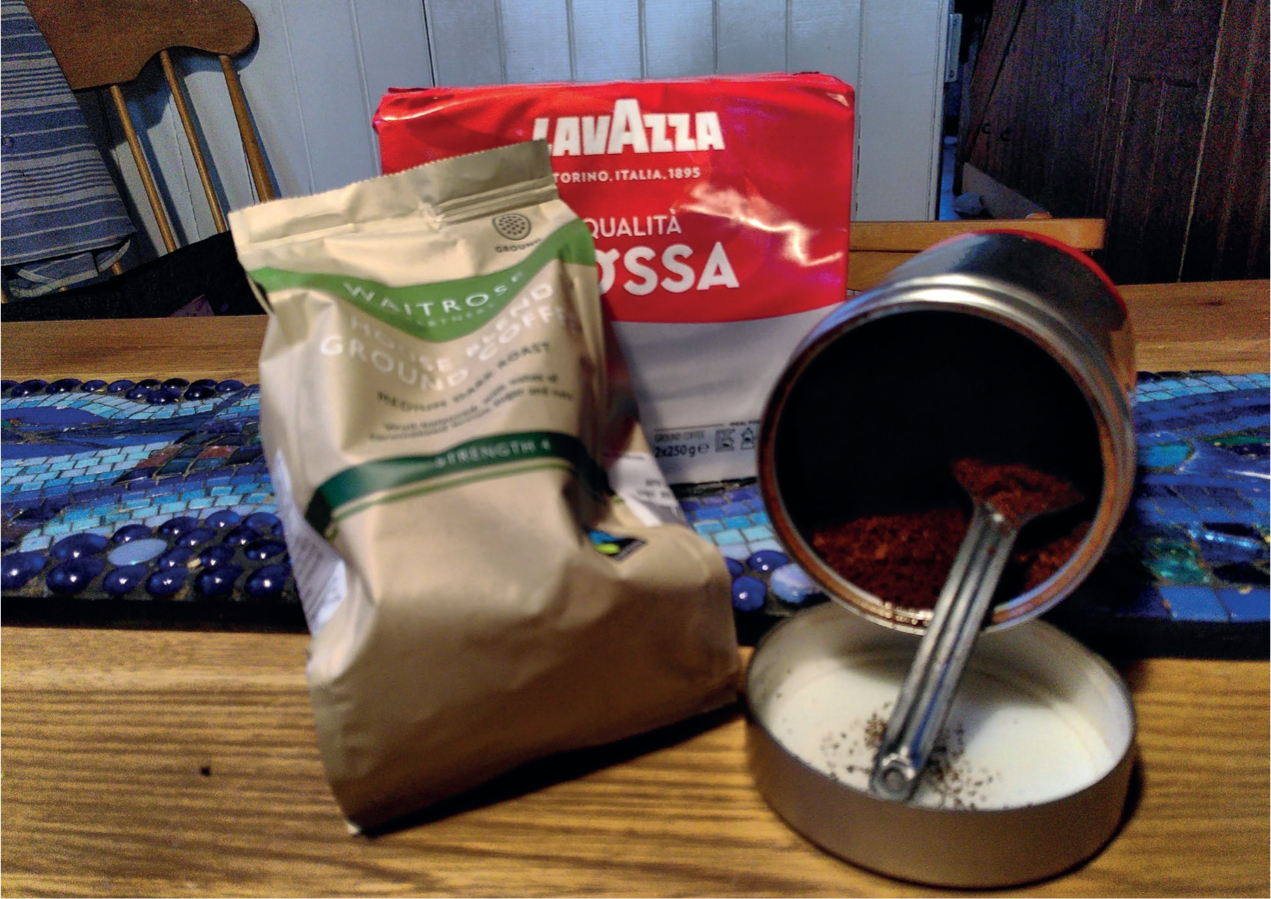
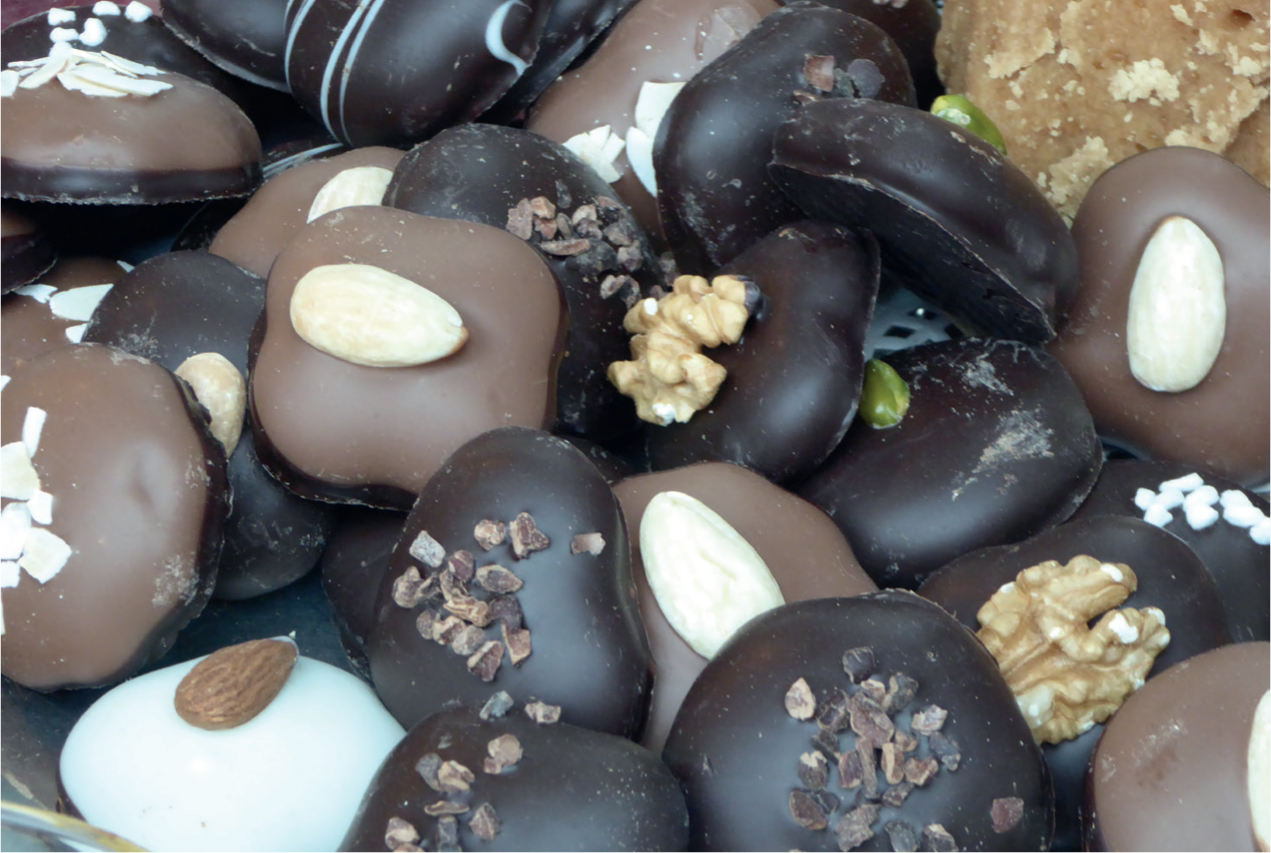
Mechanism of toxicity
Methylxanthines are central nervous system and muscle (including the myocardium) stimulants; intoxication in dogs produces a characteristic picture of central nervous system stimulation with cardiac and respiratory hyperactivity. Methylxanthines exert their effect by a variety of mechanisms. They competitively antagonise cellular adenosine receptors, resulting in central nervous system stimulation, vasoconstriction and tachycardia. They also inhibit cellular phosphodiesterase, causing an increase in cyclic adenosine monophosphate, increased release of catecholamines, increased entry of calcium and inhibition of calcium sequestration by the sarcoplasmic reticulum, causing increased muscular contractility in both skeletal and cardiac muscle (Dolder, 2013).
Clinical signs
Onset of methylxanthine toxicosis can be within 2–4 hours (Dolder, 2013), sometimes 6–12 hours (Gwaltney-Brant, 2001) and recovery make take 12–36 hours (Dolder, 2013).
Initial clinical signs include acute cardiac stimulation and central nervous system excitation with hyperactivity, restlessness, excitation, agitation, abnormal behaviour and vomiting. As these rapidly progress there may be panting, tachypnoea, tachycardia, weakness, ataxia, diuresis, diarrhoea and muscle tremors. Pyrexia, dehydration and hypokalaemia also commonly develop (Dolder, 2013). Convulsions can occur but they are rare and usually only seen in severe cases of methylxanthine toxicosis. The arrhythmias reported in more severe cases are usually ventricular premature contractions.
Fatal cases of chocolate poisoning are not common, but death can occur from caffeine usually after ingestion of a pharmaceutical product as they can contain high doses (Vig et al, 1986; MacCallum et al, 2017) (Box 2).
Treatment
Treatment of methylxanthine poisoning is aimed at gut decontamination, providing supportive care based on clinical signs, and management of central nervous system and muscle stimulation.
Induction of emesis after ingestion of a methylxanthine is best avoided in hyperactive or excitable animals; activated charcoal alone should be given. An emetic can be given if the animal is asymptomatic followed by activated charcoal. Repeated doses of activated charcoal (1–3 g/kg every 4 hours until seen in the stools) may be useful in enhancing elimination as these compounds undergo enterohepatic re-circulation.
Treatment of methylxanthine toxicosis is supportive with intravenous fluids to maintain adequate renal perfusion, correct electrolyte imbalances (Dolder, 2013) and promote excretion. An antiemetic may be given if there is persistent vomiting and the electrolytes, temperature, cardiac and respiratory function should be monitored.
Diazepam or midazolam can be given for hyperactivity or convulsions, and if ineffective a barbiturate or general anaesthesia may be required. Propranolol may be given for severe tachycardia or arrhythmias.
Ventricular arrhythmias unresponsive to a beta-blocker can be treated with lidocaine (Dolder, 2013). Atropine can be given, with caution, for bradycardia.
Conclusions
Many different types of substances found in the home can have adverse effects on cardiac and cardiovascular function. If uncontrolled, these can lead to complications of cardiac damage and tissue injury as a result of poor tissue perfusion. Management depends on the substance involved and the severity of signs.
KEY POINTS
- Many substances can cause a wide variety of effects on the heart and cardiovascular system.
- Exposure to salbutamol by puncturing an inhaler is a common cause of tachycardia in dogs.
- Beta-blockers and calcium-channel blockers can cause hypotension and arrhythmias in overdose, but severe cases are rare in dogs.
- Methylxanthines such as theobromine in chocolate and caffeine can cause central nervous system and myocardial stimulation.
- Animals with pre-existing cardiac disease or those that have taken a mixture of cardiotoxic drugs are more at risk.
- Treatment will depend on the substance involved and the severity of poisoning.