Microbiome refers to the population of microorganisms (bacteria, viruses, protozoa and fungi) that inhabit the entire gastrointestinal (GI) tract, more particularly the colon whose role it is to maintain the integrity of the intestinal mucosa and control the proliferation of pathogenic bacteria. The term microbiome refers to the total number of microorganisms and their genetic material and is contrasted from the term microbiota, which is the microbial population present in different ecosystems in the body. Alteration in the composition of the gut microbiome is called dysbiosis. It has been shown that dysbiosis predisposes to inflammatory bowel diseases such as ulcerative colitis, Crohn's disease and indeterminate colitis (Tomasello et al, 2016).
Identification of bacteria
Identification of bacteria within the canine GI tract has traditionally been achieved by culture of faecal samples. This method has, however, now been recognised as being ineffective with large numbers of bacteria remaining undetected (Suchodolski, 2011). Faecal cultures yield useful results when trying to detect specific enteropathogens such as Salmonella and Campylobacter jejuni, which is useful in a clinical setting as these bacterial infections are commonly seen in practice. However, it is thought that only 30% of GI bacteria have been able to be cultured (Fraher et al, 2012), and this does not take into consideration the rest of GI microbiome (protozoa, fungi or viruses).
A molecular method such as 16S rRNA sequencing allows bacteria to be identified in a reliable format (Figure 1). The method involves the DNA from the intestinal bacteria being extracted and the 16S rRNA gene being amplified and processed via polymerase chain reaction (PCR) using a high-throughput sequencer. Researchers sequence this short piece of DNA (the 16S rRNA), and search databases to find out if it is from a known species or one that has not yet been identified. It has been estimated that the intestinal microbiome consists of approximately 10 times more microbial cells (1012–1014) than the number of host cells, and the microbial gene pool is 100 times larger compared with the host gene pool (Suchodolski, 2011).
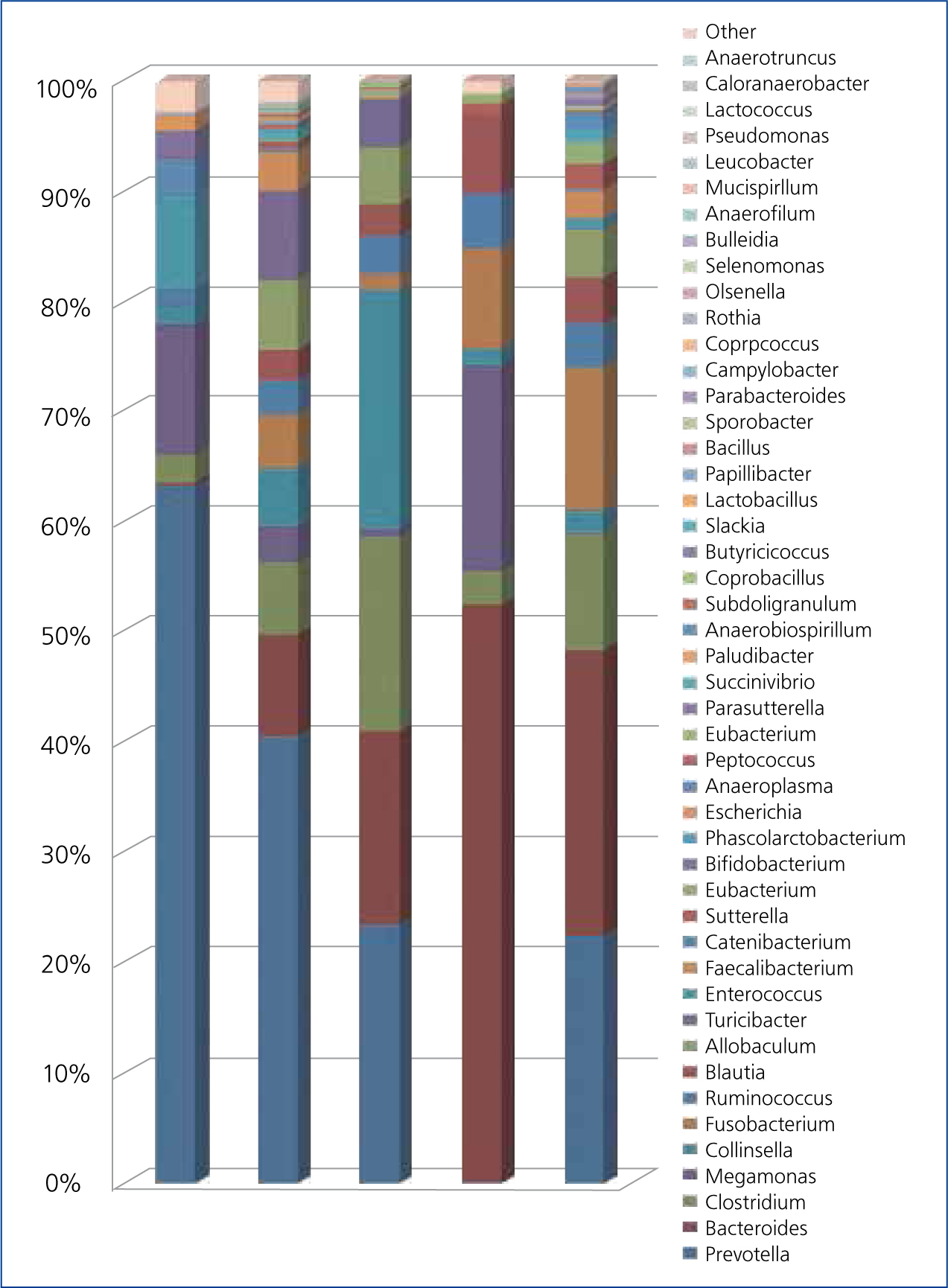
Techniques such as fluorescence in situ hybridization (FISH) utilise fluorescent probes that bind to specific parts of DNA or RNA. FISH can also be used to detect and localise specific RNA targets, and thus FISH is often used in clinical studies. If a patient is infected with a suspected pathogen, bacteria, from the patient's tissues or fluids, are typically grown on agar to determine the identity of the pathogen. Many bacteria, however, even well-known species, do not grow well under laboratory conditions. FISH can be used to detect directly the presence of the suspect on small samples of a patient's tissue. FISH can also be used to compare the genomes of two biological species, to deduce evolutionary relationships. The application of 16S rRNA gene sequence-based analysis in combination with FISH assays has enabled the discovery of invasive bacteria in the colonic mucosa of boxer dogs with granulomatous colitis (Simpson et al, 2006). Escherichia coli and Shigella have been found in heavy clusters within macrophages of these dogs. Subsequent studies in French Bulldogs has shown an association between granulomatous colitis and E. coli (Manchester et al, 2013).
Identification of the individual bacteria is important, as in these examples, however microbes are often also studied using metagenomics, a technique that reveals biological functions of an entire community. Researchers using metagenomics, for example, might take a gut sample and sequence genes from every microbe present. They can then look at the combination of genes the entire community has and use this information to describe which functions are taking place. Metagenomics does not identify individual microbes, but in some ways this is an advantage. Microbes often work together; different species in a microbe community commonly depend on metabolic products from their neighbours as resources (Delzenne et al, 2011).
Sturgeon et al (2014) presented a study looking at a small numbered population, and the differences between diet (raw and commercially fed) in 15 dogs and 10 wild wolves (eating natural diet) using sequencing of 16s rRNA gene (V4 region) PCR products. In the study, species richness of the microbiome was estimated at 76552 for dogs and 18686 for wolves. The faecal microbiota of wolves showed to be highly variable, perhaps as a reflection of the variable and inconsistency of the diet. The faecal microbiota of dogs was more conserved, with no difference in population structure between raw-and conventionally fed dogs. While diet did have an impact on the faecal microbiota of individual dogs, the faecal microbial population membership and structure is relatively conserved amongst the different feeding groups and feeding dogs raw meat does not result in development of a faecal microbiota more akin to wolves (Sturgeon et al, 2014).
What does the microbiome do?
The microbiome provides many positive effects for the host: it acts as a defensive barrier against transient bacteria; aids in nutrient breakdown and energy yield from the diet (Table 1); provides nutritional metabolites for enterocytes; and plays an important role in the regulation of the host immune system. When the different bacterial populations within the GI tract become unbalanced (dysbiosis), clinical disease can be seen. Figure 2 shows clinical disease where dysbiosis has been shown to be present.

Metabolic end products | Metabolic activities of intestinal microbiota | Effect on host health |
---|---|---|
Propionate, acetate, butyrate | Carbohydrate fermentation | Anti-inflammatory, energy source of enterocytes, regulation of intestinal motility, amelioration of leaky gut barrier |
Retinoic acid (vitamin A derivate) | Vitamin synthesis | Important for generation of peripheral regulatory T-cells |
Vitamin K2, B12, biotin, folate | Vitamin synthesis | Important co-factors for various metabolic pathways |
Ceramide | Induces degradation of sphingomyelin via alkaline sphingomyelinase | Significant role in apoptosis and in the prevention of intestinal epithelial dysplasia and tumorigenesis |
Indole | Degradation of the amino acid tryptophan | Increases epithelial-cell tight-junction resistance and attenuates indicators of inflammation |
Secondary bile acids (cholate/deoxycholate) | Deconjugation/dehydroxylation of bile acids | Intestinal fat absorption |
Taurine | Bacterial deconjugation of bile acids | Facilitates fat absorption from the GI tract, important for liver metabolism |
Oxalyl CoA decarboxylase | Degradation of oxalate through oxalyl CoA decarboxylase | Decreases in oxalate degrading enzyme associated with increased risk for calcium oxalate urolithiasis |
Ammonia | Decarboxylation, deamination of amino acids | Increases associated with encephalopathy |
D-lactate | Carbohydrate fermentation | Increases associated with encephalopathy |
Studies that assess functional aspects of the canine GI microbiota are needed. Metabolomics, the comprehensive study of small molecules present in biological samples, is an emerging method for better understanding of disease pathophysiology and host–microbe interactions, with the potential to develop novel diagnostic and/or treatment approaches (Marchesi et al, 2007; Nicholson and Lindon, 2008).
The composition of the gut microbiota has been shown to be a factor characterising differences between those populations with a disease versus a control group. Where differences have been observed, e.g. in obese versus lean individuals and diabetic versus non diabetic human patients (type 2 diabetes), the changes in the gut microbes can be reversed by dieting and related weight loss (Delzenne et al, 2011).
The microbiome has shown to cause a physical stimuli for the immune system, helping in defence against invading enteropathogens. A combination of microbial metabolites and surface molecules in the GI system activate the innate immune receptors (e.g. Toll-like receptors (TLR)) in the intestinal lining (Suchodolski and Simpson, 2013).
How can we improve the microbiome?
There are many different factors that affect how individual microbiomes differ. The gut microbial composition presents dynamic changes correlated with various factors including host genotypes, age, and external environment (stress, drugs, diet, surgery, toxins etc). Effective manipulation of the gut microbiota through diets (both long-term and short-term diet patterns), probiotics and/or prebiotics, and antibiotics has been proved, showing that there is a potential to prevent metabolic disorders, such as obesity, in many studies (Chen et al, 2014). The qualitative and quantitative changes in the intake of specific food nutrients (fatty acids, carbohydrates, micronutrients, prebiotics, probiotics), have not only consequences on the gut microbiota composition, but may modulate the expression of genes in host tissues such as the liver, adipose tissue, intestine, muscle (Delzenne et al, 2011). This may in turn drive or lessen the development of fat mass and metabolic disturbances associated with the gut barrier function and the systemic immunity. The relevance of the prebiotic or probiotic approaches in the management of obesity in humans is supported by few intervention studies in humans up to now, but the experimental data obtained with the balance of all the different nutrients help to elucidate novel potential molecular targets relating diet with gut microbes; whether these data can be extrapolated to veterinary fields is as yet unknown. The metagenomic and integrative metabolomic approaches could help elucidate which bacteria, among the trillions in human gut, or more specifically which activities/genes, could participate in the control of host energy metabolism, and could be relevant for future therapeutic developments (Delzenne et al, 2011).
There is a vast amount of work currently looking at how individual nutrients and diet ingredients can affect the GI microbiome. When the microbiome is so large, with so many variants that effect the individual populations, and these in turn effecting other populations, it can be difficult to evaluate single nutrient effects, as in many cases single nutrients will not be able to be fed in isolation.
An example of this is Spirulina, a naturally occurring blue-green algae of which there are two species (Arthrospira platensis, and A. maxima), and which has been consumed by humans as food for centuries. Spirulina has shown immune enhancing properties in animal and human studies attributed to polysaccharides acting via TLR-dependent processes. Dogs fed diets supplemented with Spirulina demonstrated enhanced immune status by showing significantly higher vaccine (rabies) response and higher levels of faecal IgA when compared with the control group (Reynolds and Satyaraj, 2014). Supplementing diets with Spirulina also resulted in significantly increased gut microflora stability in the test group. Conclusions drawn by the study authors were that dogs fed diets supplemented with Spirulina had significantly enhanced immune health and gut health. It can, perhaps, be concluded that the addition of Spirulina to the diet enhanced the microbiome, and this in turn aided in enhancing immune health and gut health in the dogs.
Antibiotics and the gut
In addition to the development of resistance, the use of antibiotics heavily disrupts the ecology of the human microbiome (i.e. the collection of cells, genes, and metabolites from the bacteria, eukaryotes, and viruses that inhabit the human body) (Langdon et al, 2016). Mounting evidence shows that antibiotics influence the function of the immune system, the body's ability to resist infection, and the capacity for processing food. It is therefore important to develop an better understanding of gut ecology and to limit the use of antibiotic compounds.
Probiotics and prebiotics
Probiotics, to replenish bacterial populations, and prebiotics, to feed and support proliferation of bacterial populations may facilitate growth of beneficial bacteria within the intestines at levels that will promote health benefits (Hamlin, 2011). However, most meta-analyses of probiotic use agree that while probiotics can be effective against a wide range of gut dysbioses, more specific data are needed to determine which probiotics are best for particular patient groups, especially as extensive variation exists between individuals in the composition of gut microbiota (Langdon et al, 2016).
Conclusion
As more technological advances are made in the identification of the constituents of the microbiome, more studies looking at cause and effect can be instigated. The multifactorial nature of the biome can make differentiating between correlation and causation difficult. The veterinary profession has only just started to look at the effects that the microbiome has on the body as a whole and a holistic approach needs to be taken when treating the animal.