Many veterinary practices have recently switched from using film-screen (plain film) radiography to computed radiography (CR). Practice managers have been relatively quick to recognise the benefits associated with using CR, many of which are financial considerations. Outwardly these two modalities appear similar, with both employing a removable cassette which has to be inserted into a processing machine. However, working with CR is not as simple as it seems. Due to the number of inherent differences between the two modalities, there are many new factors to understand in order to get the most out of a CR system and to work safely. While there is no substitute for proper training on any new piece of equipment, the purpose of this article is to explain the most important differences between film-screen radiography and CR, enabling the veterinary nurse to apply a greater degree of knowledge when using CR.
Archiving
If plain film packets are incorrectly filed it may take a long time to locate them. Films may also get lost, especially if sent in the post or if filed incorrectly. In these cases there is sometimes no alternative but to rely on the written report — if there is one — or to re-radiograph the patient. Virtually all of the problems associated with archiving plain film radiographs are overcome when using CR. The amount of space required is vastly reduced, and it takes only a few seconds to retrieve digital images from a digital archive or PACS system. Another benefit of CR is that it allows people to access the images from different locations at the same time. It is also virtually impossible to lose a CR image, and although computer systems have been known to fail on the odd occasion, regularly backing up the system up by disc can negate this concern. Being able to send or access images via the internet has the obvious advantage of convenience and time, and has led to the practice of teleradiology whereby images are reported remotely by a radiologist. This is a huge benefit for remote practices and those clinicians working out of hours.
Exposures
In order to work safely and ensure that exposures are as low as reasonably practicable (ALARP), it is important to ensure that the correct kV and mAs are being used. Traditional plain film imaging uses the ‘toast analogy’. Simply put, the more you cook it (i.e. the higher the exposure) the darker it gets. It also demonstrates what is called a narrow exposure latitude, whereby a relatively small range of exposures can be used to produce a useful image (Seeram, 2014). Plain film imaging does not allow for overexposure as the resulting images would be too dark. This built-in limiting factor is beneficial in terms of radiation protection and keeping to the ALARP principle.
CR systems demonstrate a wide exposure latitude, that is to say there is a much larger range of exposures which will result in a diagnostic image. This means that when CR is used in place of plain film, around half of the repeat radiographs will be avoided, these previously being caused by inaccurate exposure factors. This saves time, money, and in theory radiation dose to the patient.
An associated drawback is that with CR, up to a point, the signal-to-noise ratio increases with increasing exposure. In this respect higher exposures result in less noise on the radiograph, and this improves its appearance. As radiographers and radiologists prefer images with less noise there is a tendency for exposures to slowly increase over time, a phenomenon which is known as ‘exposure creep’ or ‘dose creep’ (Warren-Forward et al, 2007). Because the computer can also automatically adjust the brightness of the image, the limiting factor of overexposed films is now largely absent.
Reports differ on how much it is possible to overexpose a CR image. Carter and Veale (2010) state that exposures exceeding 200% of the ideal value will begin to lose contrast. Kowalczyk and Comer (2009) claim that CR exposures up to five times that which is needed will still be diagnostic. Exposures higher than this value may result in saturation, whereby some of the pixels will record a maximum value (black). These saturated areas will be uniformly black and will not provide any anatomical detail, even when manipulated (Seeram, 2011). This phenomenon does not always occur however. A recent study using a human chest model showed that the maximum exposure able to produce an acceptable image was obtained with a dose of 139 times that of the minimum dose needed (Ma et al, 2013). The main reason for overexposure being tolerated with CR is that images are automatically rescaled, that is to say the system adjusts the image to give a set brightness value regardless of the exposure.
While individual values will vary depending on the system used and body part examined, the primary concern here is the fact that it is possible to routinely overexpose patients and still obtain diagnostic images. Not only does this result in increased associated risks to the patient and staff (if present in the controlled area), but can decrease the lifespan of the x-ray tube which is very expensive. To address the problem CR manufacturers introduced the exposure index (EI), which is a numerical value associated with each image aimed at indicating how suitable the exposure is. It is recommended that for an appropriate exposure, this number should fall between a given set of values given by the manufacturer. Manufacturers provide a chart with the expected value range for different examinations. This system is far from perfect for a number of reasons:
- Several factors affect the EI, the most important being collimation (Baker, 2012).
- Unlike an overexposed film, the EI can simply be ignored
- Staff need to be properly trained in understanding the EI for it to work
- Different manufacturers calculate the value differently so it is hard to compare the EI directly. Standardised EI values have been recently proposed but have not yet been implemented (Seeram, 2014).
kV
For plain film imaging kV affects transmission — the degree of penetration — of the beam, and image contrast (Meredith and Massey, 1983). With CR exactly the same kV is required to penetrate the patient as with film-screen radiography, as this is independent of image receptor type (Carter and Veale, 2010). The computer's ability to automatically monitor and adjust the image's properties means that kV plays a much smaller role in determining contrast for CR.
The 10 kV rule states that increasing the kV by 10 together with halving the mAs results in a similar film density (Meredith and Massey, 1983). In film-screen radiography this is used to optimise contrast, with higher contrast being obtained at lower kV settings. Studies using CR have demonstrated that varying the kV according to the 10 kV rule had no significant effect on image quality (Al Khalifa and Brindhaban, 2004; Lanca et al, 2014). With CR, using a value of 10–20 kV higher than that employed in film-screen radiography has the benefit of significantly reducing the patient's radiation dose (Seeram, 2011).
It has been recommended that values of between 60 and 110 kV should be used to produce optimal results for CR, but values outside of this range can still be used depending on quality requirements (Carter and Veale, 2010).
mAs
mAs is not as important in terms of film density or darkness with CR as it is with film-screen radiography (Al Khalifa and Brindhaban, 2004). This is because the brightness of the image is mostly determined by the computer, in addition to having the option of being manually changed. When mAs values are too low in CR the images appear grainy or mottled, with increased noise (Figure 1). Typically this occurs where the exposure is around half that of the ideal exposure. The primary consideration with CR is therefore level of graininess or noise on the image which is considered acceptable. Ultimately this is determined by the reporting clinician who should feed back to the person performing the radiography comments concerning image quality. Where mAs settings are too high there is an excess of scattered radiation produced, which reduces the image contrast (Seeram, 2011). This is true for both radiography modalities.
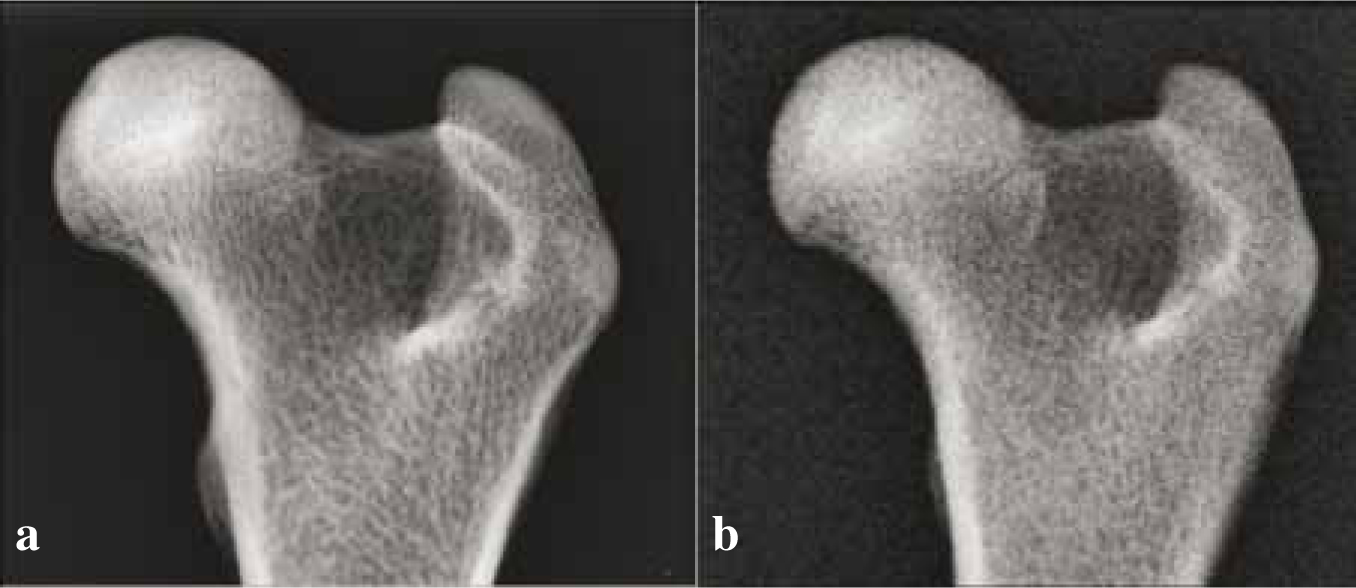
Multiple exposures
In plain film imaging it is possible to take more than one image per film. This is done by covering half of the film with lead, making an exposure and then covering the exposed half with lead and exposing the other side. The primary reason for doing this is to save money on film and processing chemicals, but it is often useful to have images of one body part together on one film for comparison.
Multiple exposures on one imaging plate (IP) are not recommended with CR. One reason for this is that CR is very sensitive to scattered radiation — see section on grids below. The second reason is that it would not save any money as images are usually not printed. In terms of image quality, multiple exposures on one IP are not going to give the best results because they are treated as one image when it comes to processing. The computer cannot optimise the appearance of more than one image per IP, and similarly when the images are post processed it is not possible to select them independently. Considering two adjacent images on one IP, one of which is too bright and the other acceptable brightness, it will only be possible to make both images brighter or darker by the same amount at the same time.
Grids
Grids present a crucial mechanism in both plain film and CR imaging by cutting out a large proportion of scattered radiation which would otherwise reach the film or IP.
CR is certainly more sensitive than film-screen radiography to low energy x-rays, such as those found in scattered radiation (Pognapang, 2005). For this reason, fogging produced by scatter is more apparent on CR (Seeram, 2011), resulting in images suffering from comparatively poorer contrast. It follows that radiographic grids may be a more important consideration for CR than for film-screen radiography.
A study by Lo et al (2009) suggested that for digital systems, when using a grid it may not be necessary to increase the exposure. This is because the greyscale brightness of the system is not directly linked to the exposure and reflects the difference between filmscreen radiography and CR in terms of mAs. However, this aspect of practice should be thoroughly investigated before any decisions are made to implement it.
Resolution
Spatial resolution is the ability to distinguish two adjacent structures as being separate from each other. In film-screen radiography the spatial resolution is governed by crystal dimensions and phosphor layer thickness (Carter and Veale, 2010). The best resolution achievable for film-screen imaging is around 10 line pairs per mm. In CR it is the phosphor layer thickness and pixel size, together with the laser scanning frequency which determine resolution. The best spatial resolution for CR is variable, but generally poorer than plain film. For example, Fuji and Philips systems operating in high quality mode achieve around four line pairs per mm (Seeram, 2011).
The poorer spatial resolution in CR is compensated for by better contrast resolution. This means that a higher range of tissue densities are seen in CR, and this increase in contrast resolution improves the image quality and compensates for the loss in spatial resolution. One suggestion to ensure the spatial resolution of CR is optimised is to use a small focal spot whenever appropriate (Pongnapang, 2005), and it is also possible to achieve higher spatial resolution by using special extremity imaging plates, such as those made by Kodak. Interestingly Agfa, Konica and Kodak systems scale the spacing of the laser scanning depending on IP size which means that using smaller-sized IPs for these manufacturers will display better resolution (Seeram, 2011).
Film speed
Film speed is governed by the dimensions of crystals in the film and screen. The speed of the filmscreen setup sets the amount of exposure needed to give the best density on the image (Seeram, 2011). As CR has no film or intensifying screens, the term ‘film speed’ is not used. Instead a different system called speed class is used based not on the receptor itself but on how much radiation is used in the first place (Carter and Veale, 2011). For CR the exposure used affects the amount of light given off by the IP, which in turn governs the speed class of the system. In general if a comparison is needed, CR imaging plates may be thought of as medium speed film (Pognapang, 2005).
Erasing imaging plates
Despite the IP being automatically erased following each exposure, this process may not be perfect and some information may still be left on the IP when it is next used (Figure 2). This is especially likely when CR images are overexposed to the point where saturation occurs (Seeram, 2011). As IPs may also be exposed to scattered radiation and background radiation over time, it is recommended that they are erased every 48 hours (Carter and Veale 2010). There is a specific function on the CR processing machine to erase scatter, which exposes the IP to a strong light source.

Time delays and heat problems
In plain film imaging, waiting before processing a film is not normally considered a problem. In CR systems the latent image is stored as electrons trapped in a higher state of excitation. When the imaging plate is read it is scanned by a laser, which releases the electrons from this state, giving off light in the process. As the process is not 100% efficient, over time some electrons will eventually fall down to their original state. Part of the useful image is therefore lost if there is a time delay between exposing the IP and processing it (Baker, 2012). This effect is more pronounced for images where a small exposure has been used.
It has also been demonstrated that heat has an adverse effect on the stored or latent image within the IP. When IPs were stored at temperatures of over 35°C a significant increase in the amount of noise was present on the resultant CR images (Al Khalifah et al, 2012).
Image annotation
As it is possible to annotate images during post processing, there is the temptation not to include an anatomical marker when taking a radiograph. Problems may arise if this is subsequently forgotten, or if the study is mistakenly sent to archive without it. This is more common when there are members of staff present who wish to see the radiographs but are unsure how to operate the image processing equipment.
Cropping tool
Although the image cropping tool gives an image which appears to be well collimated, in reality the radiographer may not have used any collimation whatsoever. There is always the temptation to be lazy with collimation with the knowledge that it can be corrected later. Conversely, when using plain film the collimation cannot be changed to appear better than it actually is, encouraging the radiographer to maintain their high standard of collimation to produce acceptable images (Baker, 2012).
By getting away with poor collimation the patient is being exposed to more radiation than is required. This in turn produces more scattered radiation, which degrades the image quality. Increased scatter also presents a hazard to any staff members present.
If the patient has been exposed to potentially harmful x-rays, the best use of all the information obtained should be ensured (Baker, 2012). Cropping the image will remove potentially useful information on the radiograph. As a result, many radiology departments now ban the use of cropping tools.
While collimation is important for both CR and film-screen radiography, it is more influential for CR in terms of image quality. The CR system is able to identify the edges of the collimation and analyses the area within it during processing. If collimation is poor this will reduce the computer's ability to optimise the image, resulting in radiographs with poorer diagnostic quality (Figure 3). While this can usually be addressed with manual post processing this is not an ideal situation; it takes more time to perform and may not be as good as the computer's automatic processing. Poor collimation in CR is also known to make the EI less accurate (Baker, 2012), making it more difficult to judge whether the exposure is correct or not.

Conclusion
Despite appearing similar, film-screen radiography and CR are different in many ways. The topics addressed in this article will enable veterinary nurses to apply a greater degree of knowledge when using CR, resulting in optimised image quality and better safety for staff and patients.
Key Points
- kV is much less influential in determining contrast for computed radiography (CR).
- mAs selection determines image noise rather than density for CR.