Surgical site infections (SSIs) are a type of iatrogenic infection occurring in wounds postoperatively, which can be a potentially life-threatening surgical complication within human and veterinary medicine (Eugster et al, 2004; Cho et al, 2007; Hemani and Lepor, 2009). SSIs can result in pain, delayed healing and wound breakdown and, because preventable, could be deemed ‘unavoidable suffering’ (Jennings and Berdory, 2010). Therefore effective SSI prevention is essential to maintain the health and welfare of surgical patients.
It is widely believed that the patient's skin is the main source of surgical wound contamination, with Staphylococci and Streptococci bacterial species most frequently cultured from veterinary SSIs (Darouiche et al, 2010; Hutchinson, 2012). These species represent the normal bacteria of the skin and are usually non-pathogenic, but can cause infection when they enter a wound (Bowers, 2012). SSIs account for 15% of human nosocomial infections, prolong hospitalisation and increase morbidity and mortality, in turn increasing the cost of surgery in humans (Durani and Leaper, 2008; Reichman and Greenberg, 2009). Veterinary SSI incidence occurs at an estimated 3% in surgical patients (Fitzpatrick and Solano, 2010; Turk et al, 2015) with livestock surgery that often occurs outside the surgical environment in situ increasing SSI risk >30% (Weaver et al, 2005; Verwilghen and Singh, 2014). Investigations into the microbiology of SSIs have demonstrated wide bacterial diversity (Owens and Stoessel, 2008; Wolcott et al., 2009; Turk et al, 2015); causal bacteria implicated include Staphylococci species (74%), with Staphylococcus aureus and coagulasenegative Staphylococci most commonly reported (Owens and Stoessel, 2008; Turk et al, 2015).
An estimated 40-60% of SSIs are avoidable (Uckay et al, 2010) and prevention of SSIs can be relatively simple. Preoperative skin preparation (PSP) with an appropriate antimicrobial is commonly practiced by veterinary professionals to decrease resident bacteria to sub-pathogenic levels, reducing SSI risk (Durani and Leaper, 2008). Prophylactic antibiotics were once recommended to prevent SSIs, however due to increasing bacterial antibiotic resistance these are no longer advised (Knights et al, 2012; Turk, 2013). The most commonly used antimicrobials in veterinary medicine are chlorhexidine gluconate (CHG) and povodine iodine (PI) (Hemani and Lepor, 2009; Bowlt and Gasson, 2013; Rutter et al, 2014). Manufacturers recommend the use of undiluted product (BCM, 2013; Animalcare, 2014), however in veterinary practice antimicrobials are commonly diluted in a solution with water and soaked swabs used for application (McHugh et al, 2011; Aspinall, 2014). SSI risk increases if the dilution is too weak, providing inadequate antibacterial action (Kampf and Kramer, 2004; Evans et al, 2009). For example, it has been demonstrated that dilutions containing less than 3% CHG reduce but do not eliminate bacteria, and are ineffective against S. aureus (Evans et al, 2009; Montevecchi et al, 2013).
CHG is an aqueous antimicrobial, effective against Gram-positive and negative bacteria, yeasts and some viruses (Reichman and Greenberg, 2009; Macias et al, 2013). It has bactericidal action by disturbing bacterial cell membranes and increasing cell wall permeability, facilitating bacteriolysis (Karki and Cheng, 2012; Edmiston et al, 2013). CHG binds to the surface of the skin to produce a residual effect, enabling lasting antibacterial activity long after application (Anderson et al, 2010; Sogawa et al, 2010). It is thought that CHG products are also able to delay the recolonisation of resident bacteria (Rutter et al, 2014). PI is another popular topical antimicrobial choice, as it is broadspectrum and safe for application to mucous membranes (Hemani and Lepor, 2009). It is a water-soluble complex of iodine and a carrier, polyvinylpyrrolidone, which acts as a reservoir of the active ingredient free iodine (Anderson et al, 2010). PI is an aqueous-based iodophor, whose antimicrobial properties relate to its ability to destroy bacterial proteins and DNA (Hemani and Lepor, 2009). PI is considered to have some level of persistence, although comparatively it is thought that CHG has a longer residual effect (Sogawa et al, 2010; Pelligand, 2012). Few studies have compared the efficacy of PI with other antiseptics; however cultures from surgical sites 30 minutes post-application have suggested that CHG is more effective than PI (Culligan et al, 2005). Other studies have also reported a superior effect of CHG when compared with PI, with lowered colony counts at the surgical site after PSP (Art, 2007; Macias et al, 2013). Interestingly, limited research has evaluated the synergy between antimicrobials; synergism between CHG and PI has been reported but further research into the potential combination of antimicrobials for SSI reduction in practice is needed (Anderson et al, 2010).
Resistance to both CHG and PI has been recorded, therefore it is becoming increasingly important to establish novel, safe alternatives (Reichman and Greenberg, 2009; Anita et al, 2015). There has been interest in the antimicrobial effects of tea for many years; recent in vitro and in vivo studies into Camellia sinensis (green tea: GT) have provided a better understanding of its antimicrobial properties (Kumar et al, 2012; Sharma et al, 2012). GT contains medically important compounds including polyphenols (Silva et al, 2013) and flavonoids (Reygaert, 2014). Flavonoids, for example catechins, constitute approximately 30–40% of dry leaf weight and are thought to account for GT's antimicrobial properties (Almajano et al, 2008); Reygaert, 2014). Catechins have a direct antibacterial effect by binding to the lipid bilayer of bacterial cell membranes, thus causing damage and preventing bacteria forming biofilms (Reygaert, 2014). Fatty acids are a major component of the phospholipid bilayer of the cell membrane and there is evidence that GT catechins, especially epigallocatechin-3-gallate (ECGC), can also inhibit enzymes that are required for fatty acid synthesis (Singh et al, 2011; Wang and Ma, 2013) and DNA replication, enabling them to possess potent antibacterial activity (Grandišar et al, 2007).
Mechanisms of action differ between antimicrobials; CHG affects cell membranes, PI targets intracellular molecules and GT uses a combination of both mechanisms (Durani and Leaper, 2008; Reygaert, 2014). Research to directly compare the efficacies of such antimicrobials has not yet been conducted, therefore this study aimed to provide a framework for further research into the potential use of C. sinensis (GT) in veterinary PSP, by comparing its efficacy at preventing bacterial growth of Staphylococci and Streptococci species to that of CHG and PI in vitro.
Materials and methods
The Kirby-Bauer disk diffusion method (disk diffusion) was used to determine the in vitro susceptibility of S. aureus (ATCC 25923), Staphylococcus intermedius (ATCC 29663), Streptococcus uberis (ATCC 9927) and Streptococcus pyogenes (ATCC 19615) to dilutions of 4% CHG, 10% PI and GT (Hudzicki, 2013; Vetbact, 2015).
Commercially available CHG (Hibiscrub™, BCM Ltd) and PI (Vetasept™ PI antiseptic) (Animalcare) products contain 4% chorlohexidine gluconate and 10% PI, the active ingredient respectively. Based on previous research, 10%, 5%, 2.5% and 1.25% dilutions of CHG, PI and GT were prepared (Yassen et al, 2011). A 0% dilution (distilled water) was used as a control. Fresh dilutions were prepared daily to avoid degradation. The appropriate measure of undiluted CHG or PI was mixed with sterile 0.9% saline as a buffer to obtain 1 ml of the required dilution; sufficient volume to impregnate six filter disks per plate. For example, the 10% dilution was prepared using 0.9 ml sterile saline mixed with 0.1 ml CHG in a sterile Eppendorf tube.
Dilutions of GT were prepared using dried GT leaves (Clipper®) and distilled water. For the 10% dilution, 10 g loose dried GT leaves was added to 90 ml water; this was repeated for each dilution, for example 5 g tea added to 95 ml water for a 5% dilution. Following the manufacturer's recommendations, distilled water was boiled, measured and left to cool for 1 minute before pouring over the leaves. The solution was stirred once and left to infuse for 2 minutes. Then 1.5 ml of the liquid was decanted into a sterile Eppendorf tube using an automatic pipette with a sterile tip, and allowed to cool before application to prevent heat destroying the bacteria. The process was repeated for each dilution of tea.
Inoculation and application of antimicrobials
Sixteen plates were prepared for inoculation with each bacterium: S. aureus, S. intermedius, S. uberis and S. pyogenes. The lawn method of inoculation was used to inoculate Mueller-Hinton agar plates to promote an even layer of bacterial growth, useful for susceptibility testing (Parija, 2009; Driscoll et al, 2012) (Figure 1).
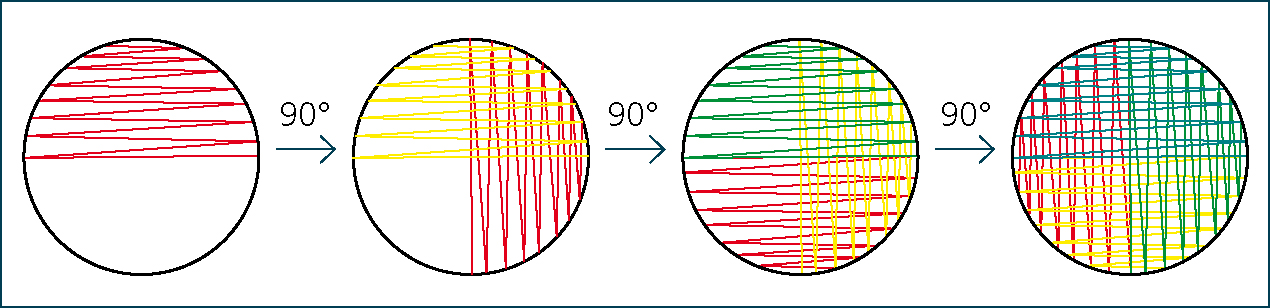
Application of filter disks and antimicrobials
Following the Kirby-Bauer disk diffusion test protocol (Eucast, 2015), six filter disks were applied to the surface of the inoculated agar of each plate and pressed down gently with forceps to ensure complete contact with the agar. The disks were spaced evenly to allow visualisation of clear zones of inhibition (ZOI). Each filter disk represented a repeat therefore there were six repeats per antimicrobial dilution per bacterium (Parija, 2009). An automatic pipette was then used to apply 10 μl of antimicrobial to each filter disk, avoiding surface pooling which could cause inaccurate results. Plates were then incubated at 37°C for 20 hours prior to collection of results (Hudzicki, 2013).
Data collection
The diameters of ZOIs (DZOIs) were measured in millimetres using Vernier callipers. In the case that two ZOIs overlapped, the radius of the zone was measured and multiplied by two to produce an estimate of the diameter. If there was no ZOI the bacterium was considered resistant, with DZOIs recorded as 0 mm (Hudzicki, 2013).
Data analysis
The mean and standard deviation for DZOI for the six filter disks (n=92) on each plate (n=16) for each bacterium were calculated using Microsoft Excel, version 2013. Data were analysed using Statiscal Package for the Social Sciences, version 20. A series of Kruskal-Wallis analyses were undertaken to determine the presence of a difference between the DZOI, with increased diameters representing enhanced efficacy, for each of the four bacterial species, for dilutions within each antimicrobial, between the antibacterials and to compare synthetic (CHG and PI)) versus natural products (GT)(Field, 2009). Subsequent post-hoc testing was completed using Mann-Whitney U tests with a bonferroni adjustment applied to the post-hoc alpha value due to the inclusion of repeated groups, to prevent the occurrence of Type I errors (revised significance level: p<0.016) (Field, 2009).
Results
All dilutions of CHG were effective at reducing bacterial growth in all species, demonstrated by clear zones of bacterial inhibition around the disks, and were larger than the other antimicrobials; dilutions of PI and GT were less effective at bacterial reduction, demonstrating largely similar ZOI (Figure 2). Significant differences were found between mean DZOIs for the three antimicrobials (p=0.0001). Subsequent post-hoc testing indicated CHG (mean ZOI: 24.02 mm±2.05) was more effective than both PI (mean ZOI: 4.46±1.35 mm) and GT (mean ZOI: 2.90±2.60 mm). No significant difference in DZOI was found between PI and GT (p=0.022).

CHG versus PI
CHG inhibited growth of all species of bacteria tested; in contrast, only 10% PI mimicked this action. Mean DZOIs for CHG across all dilutions (24.04±2.05 mm) were larger than PI DZOIs (4.46±1.35 mm), with the ZOI of 1.25% CHG 49% larger than 10% PI for all bacteria spp.. Differences were exposed between the mean DZOI for the different dilutions of CHG and PI for all bacteria tested (p<0.0001) (Table 1). Enhanced efficacy was found for all dilutions of CHG compared with all dilutions of PI for all bacteria (p<0.001) (Figure 2). Further significant differences in antimicrobial efficacy were exposed between the mean DZOIs of the variable dilutions of PI (p<0.0001); DZOI diameter expanded with increased concentration (10%: 10.32±0.94 mm; 5%: 4.44±3.65 mm; 2.5%: 1.37±2.38 mm) (Table 1). In contrast, no significant difference in CHG efficacy was found between all dilutions, across all bacteria (p>0.05).
Dilution of PI | Result |
---|---|
10% > 5% | p=0.0001 |
10% > 2.5% | p=0.0001 |
10% > 1.25% | p=0.0001 |
5% > 2.5% | p=0.0001 |
5% > 1.25% | p=0.0001 |
2.5% and 1.25% | p=0.039 |
Zones of inhibition for each dilution of povodine iodine (PI) were tested to compare efficacy of the different dilutions across all bacterial species evaluated: Staphylococcus aureus, Staphylococcus intermedius, Streptococcus uberis and Streptococcus pyogenes; ‘>’ indicates increased efficacy, i.e. reduced diameters for zones of inhibition. |
Dilutions of GT
GT appeared effective against three of the four bacteria tested; 10%, 5% and 2.5% dilutions of GT were effective against S. pyogenes, in contrast only 10% GT was effective against S. aureus and S. uberis. S. intermedius was unaffected by the presence of GT, with no ZOI evident for any dilution. The 10% dilution was most effective (mean DZOI: 7.17±4.23 mm), whereas 2.5% produced the smallest effect (mean DZOI: 2.08±3.61 mm) and 1.25% GT was ineffective against all bacteria (Table 2). No differences in efficacy (p>0.05) were found between the dilutions of GT when used on S. intermedius (Table 2). Efficacy did vary significantly (p<0.02) between dilutions against the remaining bacteria: the 10% dilution of GT exhibited an antimicrobial action against S. aureus and S. uberis, however all dilutions of GT >2.50% prohibited growth of S. pyogenes (Table 3).
Dilution rate | Staphylococcus aureus Mean ZOI (mm) | Staphylococcus intermedius Mean ZOI (mm) | Streptococcus uberis Mean ZOI (mm) | Streptococcus pyogenes Mean ZOI (mm) | Mean ZOI across all bacterial species ± standard deviation |
---|---|---|---|---|---|
10% | 9.67 | 0 | 8.25 | 10.75 | 7.16±4.23 |
5% | 0 | 0 | 0 | 9.33 | 2.33±4.04 |
2.5% | 0 | 0 | 0 | 8.33 | 2.08±3.61 |
1.25% | 0 | 0 | 0 | 0 | 0±0 |
Mean zones of inhibition were measured for each bacteria species tested and an average overall zone of inhibition was calculated. |
Dilution rate | Staphylococcus aureus | Streptococcus uberis | Streptococcus pyogenes |
---|---|---|---|
10% > 5% | p=0.002 | p=0.015 | p=0.009 |
10% > 2.5% | p=0.002 | p=0.015 | p=0.002 |
10% > 1.25% | p=0.002 | p=0.015 | p=0.002 |
5% > 2.5% | p=1.000 | p=1.000 | p=0.015 |
5% > 1.25% | p=1.000 | p=1.000 | p=0.002 |
2.5% > 1.25% | p=1.000 | p=1.000 | p=0.002 |
Zones of inhibition for each dilution of green tea were tested to compare efficacy of the different dilutions across the three bacterial species were growth was inhibited: Staphylococcus aureus, Streptococcus uberis and Streptococcus pyogenes; ‘>’ indicates increased efficacy i.e. reduced diameters for zones of inhibition. |
Synthetic versus natural antimicrobials
The synthetic antimicrobials (CHG and PI) produced a significantly higher mean DZOI (p<0.0001) for all bacteria species (mean DZOI: 14.24±1.59 mm) when compared with GT (mean DZOI: 2.90±2.60 mm) (Figure 3). Interestingly, no significant difference (p>0.05) was found between mean DZOIs of PI and GT, with the exception of S. intermedius (where GT was ineffective at all dilutions; p<0.001). However CHG was significantly more effective than GT against all bacteria tested (p<0.0001).
Discussion
CHG proved the superior antimicrobial against the bacterial species tested in this study; CHG has previously been reported to produce a superior effect in comparison to other antimicrobials including PI, supporting the observed results (Darouiche et al, 2010; Jarral et al, 2011; Kunkle et al, 2014). Manufacturers' guidelines indicate that Vetasept (10% PI) and Hibiscrub (4% CHG) are recommended for undiluted use during PSP (Animalcare, 2014, BCM, 2013), as there are no clear guidelines and conflicting recommendations for PSP with CHG within the veterinary literature it could be assumed that it could be used diluted or undiluted in veterinary practice (BCM, 2013; Aspinall, 2014). The antimicrobial action of the dilutions tested here suggest this assumption would not enhance the risk of SSIs. In contrast, the application of diluted PI reduced its antimicrobial action and could increase the risk of SSIs. In veterinary practice both PI and CHG are used diluted, perhaps at insufficient concentrations, and applied using soaked swabs (McHugh et al, 2012; Aspinall, 2014), often followed by application of iodophor alcohol (surgical spirit). Alcohol has been shown to have a short-acting broad spectrum antimicrobial activity which complements the action of CHG (Hemani and Lepor, 2009). A dilution ratio of 50:50 CHG solution: water followed by a final surgical spirit application is therefore recommended for use for surgical site preparation in the veterinary patient (Hemani and Lepor, 2009). However further investigations into antimicrobial dilutions used in vivo and incidence of SSIs are warranted to determine the impact of common practices on SSI risk.
The comparison of relative dilutions of CHG and PI demonstrated that CHG produced a far superior effect when used on the bacteria tested, indicating that CHG should be the preferred choice for PSP in most surgeries. The superior antimicrobial action of CHG against the bacteria tested in this study, may be related to CHG's 99% bacterial kill rate within 30 seconds of application coupled with its residual activity; this may have initially eliminated the bacteria in direct contact with the antimicrobial as it diffused through the agar, producing clear zones around the disks as fewer bacteria were available to colonise (Orpet and Welsh, 2010; Macias et al, 2013). Despite evidence of diffusion through the agar, PI and GT did not produce a comparative residual effect; however it is possible some regrowth occurred during incubation. It is possible that PI could produce a superior effect when used in vivo, therefore further research to determine this is indicated (Osuna et al, 1992).
It is commonly perceived that PI is effective at reducing resident skin bacteria so is often used in veterinary practice, whereas the results obtained here suggest poor efficacy (Bowers, 2012). These results may indicate an over-reliance on PI for SSI reduction in the veterinary industry (Hedalgo and Dominguez, 2001; Hemani and Lepor, 2009; Bowlt and Gasson, 2013). This is particularly true for large animal surgery where PI is often used, especially during field procedures, despite having been demonstrated to be less effective than CHG in these species (Desrochers et al, 1996, Wilson et al, 2011). The results suggest that CHG would be recommended as the superior produce for PSP, however as its use is contraindicated in certain surgeries such as ophthalmic, aural or neurosurgery, PI still plays an important role in SSI prevention in some cases (Denton, 2001). These results could assist veterinary professionals make an informed decision on the best antimicrobial product for use during general PSP.
pH can influence the antimicrobial activity of the products tested; PI is active at pH 3 to 5.5 whereas Mueler-Hinton agar is pH 7.4±0.2, which could account for the reduced performance observed (Atlas and Snyder, 2006; Animalcare, 2014). It has been found that normal animal skin is approximately pH 6; although more acidic than MuellerHinton, this is still outside the active pH range of PI, which could adversely impact its efficacy in vivo (Meyer and Neurand, 1991). No recommendations for pH are available for CHG. Contact times are another important factor in the determination of the efficacy of antimicrobials, with increasing time related to superior efficacy (Koburger et al, 2009). However Evans et al (2009) found that the majority of veterinary nurses were unaware of contact times used during PSP, suggesting that educating veterinary professionals in the appropriate use of antimicrobials may be an equally important factor in future SSI reduction.
Efficacy of GT
Demand for natural products is growing in many industries, with particular interest in natural pharmaceuticals and an emphasis on antimicrobials due to increasing bacterial resistance (Sharif et al, 2014; Ling et al, 2015). However, GT was not found to have comparative antimicrobial properties to CHG and PI. Ten percent GT was the most effective dilution tested (mean DZOI 7.16±4.23 mm) and 2.5% the least effective (mean: 2.08±3.61 mm). Growth within the GT ZOIs was evident, suggesting some concentrations were not sufficient to clear all bacteria. It is probable that the higher the concentration of GT, the more numerous the water-soluble catechins present. As these possess antibacterial properties, a direct correlation would be expected between increasing concentrations of GT and antibacterial activity (Cushnie and Lamb, 2005). A manufactured antimicrobial based on GT may be more appropriate for PSP than the infused preparation used here, to ensure adequate concentrations of catechins are present. Synergistic effects have also been observed when GT and antimicrobials are used concurrently. Therefore the addition of GT catechins to skin preparation products such as CHG and PI could enhance their antibacterial activity (Tiwari et al, 2005; Archana and Abraham, 2011; Reygaert, 2014). Further research investigating GT used solely or in combination with other antimicrobials for SSI prevention is therefore needed.
A sample of bacteria were selected here to represent normal skin flora, however in reality these would contain a more diverse range of bacterial species (Grice and Segre, 2011). Therefore to fully determine the efficacy of an antimicrobial for skin preparation it should be tested on all bacteria that might be present in vivo. Future research could culture swabs taken directly from animal skin to test the efficacies of antimicrobials. It is also possible that in vitro results may not directly correlate with in vivo efficacy (Hardy Diagnostics, 2015), consequently the conclusions drawn from this study should be interpreted with caution.
Conclusions
The results of this study advocate the use of CHG as the most effective topical antimicrobial for surgical preparation of the veterinary patient. While GT was shown to exhibit some antibacterial action against common skin bacteria, this was inferior to veterinary antimicrobials currently used in PSP. Therefore GT in the formulation tested is not recommended for use as a veterinary antimicrobial.