An electrolyte is a substance that dissociates into ions in solution and is capable of transporting an electric charge. Electrolytes can be positively or negatively charged and are balanced within the body. Positively charged ions are cations and negatively charged are anions.
The major electrolytes in the body include:
- Sodium (Na)
- Chloride (Cl)
- Potassium (K)
- Calcium (Ca)
- Phosphate (PO4)
- Magnesium (Mg).
Electrolytes help regulate fluid balance, nerve and muscle function and maintain blood pH. Concentrations of electrolytes are closely controlled by the action of different hormones and the kidneys. Derangements can affect many body functions including cardiac, skeletal muscle function and the nervous system. The first part of this two part series will look at sodium, chloride and potassium.
Sodium
Sodium is the major body extracellular cation and maintains the osmolality of extracellular fluid. Normal values are around 140 milliequivalents (mEq)/litre in extracellular fluid and 10–12 mEq/litre in intracellular fluid (Scalf, 2014).
Most sodium concentration disturbances are caused by abnormalities in water handling rather than a change in the number of sodium molecules.
The kidney is responsible for 99.5% of sodium filtered and most of the reabsorption occurs via the sodium/potassium ATPase pumps in the proximal convoluted tubule. Renal receptors detect changes in kidney perfusion which leads to activation of the renin angiotensin aldosterone system (RAAS). Aldosterone then acts on the kidney to reabsorb sodium in the distal convoluted tubule.
Sodium is classed as an effective osmole, which is a substance that is dissolved in water but does not freely cross cell membranes and exerts an osmotic force. When sodium increases in the extracellular space it increases the effective osmolality, causing water to leave cells along the osmotic gradient resulting in cell shrinkage. If free water is added to the interstitium, cells swell as water moves along the osmotic gradient to achieve water balance.
Hypernatraemia
Hypernatraemia is defined as a plasma or serum sodium concentration greater than the reference interval (Table 1) (Burkitt Creedon, 2015). Aetiology is usually as a result of excessive free water or hypotonic water loss and rarely as a result of excess sodium intake or retention (Table 2) (Burkitt Creedon, 2015).
Table 1. Normal sodium values
Canine | 140–150 | mEq/litre |
Feline | 146–157 | mEq/litre |
Table 2. Causes of hypernatraemia
Free water/pure water loss as a result of: Insensible water loss through panting, fever, seizuresDiabetes insipidus (central or nephrogenic)Inadequate water intake; lack of access or hypothalamic disorders such as primary hypodipsia/adipsia |
Hypotonic water loss as a result of: VomitingDiarrhoeaRenal failure (acute or chronic)Diuretic administrationPost obstructive diuresisDiabetes mellitusThird space losses in peritonitis or pancreatitis |
Sodium gain: Through ingestion or iatrogenic from hypertonic saline, sodium bicarbonate use or sodium phosphate enema administration |
Sodium gain is rare but can occur as a result of ingestion of high sodium products (salt dough, beef jerky, salami or sea water), hyperaldosteronism or can be iatrogenic (Burkitt Creedon, 2015).
Clinical signs of hypernatraemia
Neurological signs are usually seen in acute or severe cases of hypernatraemia (sodium >180 mEq/litre) and include disorientation, head pressing, ataxia, behaviour changes, obtundation, seizures, coma and can even lead to death (Burkitt Creedon, 2015). Signs are a result of movement of water out of cerebral cells and can lead to focal haemorrhage caused by rupture of cerebral vessels if the movement of water occurs rapidly.
If sodium levels rise slowly, the body provides protective measures. Free water starts to move out of the intracellular and into the extracellular space leading to decreased cell volume. In early stages as neuronal water is lost, lowered interstitial hydraulic pressure draws fluid from the cerebro spinal fluid (CSF) into the brain interstitium. As plasma osmolality rises, sodium and chloride ions move from the CSF into cerebral tissue, which helps to minimise brain volume loss. Within 24 hours neurons begin to accumulate organic solutes to increase intracellular osmolality and shift lost water back into the intracellular space.
Organic solutes are idiogenic osmoles or osmolytes and consist of glutamate, inositol and some amino acids like glutamine. Generation starts within a few hours of cell volume loss, but may take as long as 2–7 days for full compensation to occur (Burkitt Creedon, 2015).
Treatment of hypernatraemia
In hypernatraemia cases over 170 mEq/l that have been present for longer than 24 hours sodium levels should be corrected over a period of 48–72 hours (Goldkamp Schaer, 2007; Scalf, 2014). In chronic cases sodium levels may be required to be lowered safely over longer periods.
In cases of mild to moderate hypernatraemia (<180 mEq/litre), sodium should be decreased at a rate of no more than 1 mEq/litre/hour, and in cases of severe hypernatraemia (>180 mEq/litre) sodium should cautiously be decreased at a rate no more than 0.5 mEq/litre/hour to prevent cellular swelling (Burkitt Creedon, 2015). Idiogenic osmoles are broken down slowly, so rapid drops in sodium in chronic cases can result in neuronal oedema as a result of free water movement back into the intracellular space.
When treating hypernatraemia the goal is to replace the free water deficit and restore electrolyte balance.
Free water deficit can be calculated as follows : ( [ CURRENT SODIUM ÷ NORMAL SODIUM ] − 1 ) X ( 0 . 6 X BODYWEIGHT IN KG )
The volume of free water calculated (Silverstein and Hopper, 2015) is administered over a number of hours to safely drop sodium levels at the recommended rate. Dextrose 5% water (D5W) is used to deliver free water, however if the patient has concurrent losses (dehydration/hypovolaemia) a combination of D5W and isotonic fluids as separate infusions can be administered (Goldkamp Schaer, 2007).
Continuous monitoring for mentation or central nervous system (CNS) changes is required alongside frequent sodium level tests. Water measurement charts should be used in those that are able to drink so this can be accounted for in free water replacement therapy.
A long-stay catheter or central line may be considered in cases of hypernatraemia to minimise patient stress during serial sampling and facilitating multiple fluid infusions to be administered. Consideration should be taken in patients exhibiting neurological signs because elevating the patient's neck and jugular vein can increase intracranial pressure (Brainard, 2011).
Complications of treatment are primarily cerebral oedema. Clinical signs include head pressing, obtundation, seizures, ataxia, behaviour changes and coma. If any of these clinical signs occur during treatment, then any fluid therapy containing a lower sodium concentration than the patient's should be ceased and access to water withdrawn. Blood samples to check sodium levels should be performed to confirm if CNS signs are a result of a reduction in sodium levels or if they are a symptom of worsening hypernatraemia. Treatment for cerebral oedema is 0.5–1 g/kg intravenous mannitol given via a filter over 20–30 minutes. If mannitol is not available then a dose of 2–5 ml/kg of hypertonic saline can be given, however it is not ideal because of the high level of sodium within the fluid. Hypertonic saline can cause vasodilation if administered too rapidly so should ideally be given over 20–30 minutes (Burkitt Creedon, 2015).
Hyponatraemia
Hyponatraemia is defined as sodium concentration below the reference range and is a result of free water retention or gain with the addition of decreased water excretion. The common cause is decreased effective circulating volume which causes anti-diuretic hormone (ADH) release and increased water intake in defence of decreased intravascular volume (Table 3).
Table 3. Causes of hyponatraemia
Congestive heart failureExcessive gastrointestinal lossExcessive urinary loss (renal failure)Body cavity effusions/oedematous statesSyndrome of inappropriate anti diuretic hormone (SIADH)HypoadrenocorticismDiureticsHyperglycaemiaHyperlipidaemiaHyperproteinaemia |
In cases of hyponatraemia with atypical Addison's where aldosterone levels are normal, patients have an increase in ADH release secondary to low cortisol levels, which results in water retention. Congestive heart failure patients actually have an increase in total body sodium because of activation of the RAAS, yet are hyponatraemic as a result of increased water retention secondary to excess sodium.
Clinical signs and pathophysiology of hyponatraemia
If hyponatraemia occurs rapidly or is severe (<120 mEq/litre), signs such as head pressing, obtundation, seizures, coma or even death can occur. All cells within the body that have sodium/potassium ATPase pumps swell as a result of water moving into relatively hyperosmolar cells from what is now hypo-osmolar extracellular fluid. Neurons are clinically the least tolerant to change in cell volume (Burkitt Creedon 2015).
CNS oedema increases intracellular hydrostatic pressure which enhances fluid movement into the CSF, and flows out of the cranium back to the venous circulation. Swollen neurons expel solutes (sodium, potassium and organic osmolytes) to decrease their osmolality and encourage water loss. However ions are expelled rapidly but osmolytes take hours to days to expel, which is why clinical signs vary depending on severity and rate of change.
Pseudohyponatraemia is a condition that occurs in hyperglycaemia and in hyperlipidaemia/hyperproteinaemia where large amounts of protein or lipid interfere with laboratory machines resulting in falsely low sodium levels being recorded (Scalf 14). In cases of hyperglycaemia excess glucose molecules result in sodium dilution due to an increase in fluid in the extra cellular space. In these cases no treatment is required as the sodium concentration will increase as glucose levels return to normal.
A calculation can be used to determine the corrected sodium level in these cases (Burkitt Creedon, 2015).
SODIUM CORRECTED = SODIUM ( MEASURED ) + 1 . 6 ( [ MEASURED GLUCOSE − NORMAL GLUCOSE ] ÷ 100 )
Treatment of hyponatraemia
In normovolaemic, symptomatic patients treatment is required when sodium levels are <120 mEq/litre. The aim is to raise the patient's sodium levels to subside the clinical signs without resulting in further complications. Calculating the sodium deficit is required using the calculation below (Burkitt Creedon, 2015).
Target sodium should be no more than 10–15% of the current sodium level.
SODIUM DEFICIT = ( TARGET SODIUM − PATIENT SODIUM ) X ( 0 . 6 X BODYWEIGHT IN KG )
The goal is to raise sodium levels by 10–12 mEq/litre during the first 24 hours, and by no more than 18 mEq/litre in 48 hours. The limitation of 10 mEq/litre in the first 24 hour period is more critical than a rate over a specific period within a day; however, it equates to around 0.5 mEq/litre/hour over 24 hours (Scalf, 2014; Burkitt Creedon, 2015).
Sodium chloride (Nacl) 0.9% constant rate infusions and diuretic therapy are used to raise sodium levels. If adjustment is not achieved via this method, then hypertonic saline as a 3% solution (Burkitt Creedon, 2015) or as an admixture with isotonic solutions can be used.
Complications of correction can be severe and in chronic cases the treatment can be more dangerous than the imbalance itself. Osmotic demyelination/myelinosis can occur because of neuronal shrinkage. As water moves out of the neuron during correction it can shrink away from the myelin sheath, which can cause irreversible damage (Burkitt Creedon, 2015).
Clinical signs (ataxia, dysphagia, obtundation and paresis) can manifest themselves days after treatment. Complications usually occur when corrections exceed the recommended daily rate.
Chloride
Chloride accounts for around two thirds of the anions in extracellular fluid and has a major influence on acid–base balance. It is produced from gastric juice containing large amounts of chloride, and reabsorption occurs in the jejunum and colon. Chloride is concentrated and filtered in the glomerulus and is linked to active and passive reabsorption of sodium. Changes in free water and sodium can alter chloride concentration in the body so determination of artefactual chloride changes may need to be calculated in patients with free water and sodium changes using the calculation below (Scalf, 2014):
CORRECTED CHLORIDE = MEASURED Cl X ( NORMAL Na ÷ MEASURED Na )
Normal plasma concentrations are 110–112 mEq/litre (Scalf, 2014).
There are no clinical signs of hypo/hyperchloraemia, but the resultant change in acid–base may cause complications. Treatment of the underlying cause usually corrects the imbalance.
Examples of excessive loss of chloride include: vomiting, pyloric outflow obstruction, gastrointestinal disease, hyperadrenocorticism, loop diuretic administration, sodium bicarbonate or fluid administration (Scalf, 2014).
Causes of hyperchloraemia are renal disease, renal tubular acidosis, diarrhoea, diabetic ketoacidosis (kidneys excrete ketones instead of chloride), administration of Nacl 0.9% with potassium chloride supplementation in large quantities, hypoadrenocorticism, chronic respiratory issues and long-term use of carbonic anhydrase inhibitors (for glaucoma) (Scalf, 2014).
Potassium
Potassium is the major intracellular cation with 98–99% of potassium being in the intracellular compartment, most of which lies in skeletal muscle cells. Normal serum levels are around 3.5–5.5 mEq/litre (Scalf, 2014; Riordan and Schaer, 2015), but do not reflect the whole body content. Potassium is very important for the maintenance of normal membrane resting potential and essential for neuromuscular function.
The majority (90–95%) of potassium is excreted through the kidney with the remaining 5–10% excreted by the colon. Potassium is mainly absorbed (∼70%) in the proximal convoluted tubule.
Movement of dietary potassium is facilitated by insulin and catecholamines. Cellular concentrations will decrease in skeletal muscle first to protect brain and cardiac cells and maintain function.
Pseudohyperkalaemia can occur in the presence of cell destruction (tumour lysis syndrome, haemolysis, rhabdomyolysis, thrombocytosis, leucocytosis) which causes intracellular potassium release. Akitas and other Japanese breeds have erythrocytes that have functional sodium-potassium ATPase activity in their membrane, which leads to pseudohyperkalaemia as a result of in vitro haemolysis in blood samples (Riordan and Schaer, 2015).
Hyperkalaemia
Hyperkalaemia is defined as a serum potassium concentration that exceeds 5.5 mEq/litre (Riordan and Schaer, 2015). Hyperkalaemia is uncommon in patients with normal renal function where insulin redistributes potassium into the cells and the kidneys can excrete the excess. Hyperkalaemia because of intake of potassium is unlikely, unless the patient is receiving a rapid or high concentration of potassium containing fluid (Table 4).
Table 4. Causes of hyperkalaemia
Diabetes mellitus with ketoacidosis or hyperosmololityAnuric/oliguric acute renal failureUrethral obstructionUrinary bladder ruptureHypoadrenocorticismSome GI diseases (salmonellosis, perforated duodenum)Chylothorax managed by mechanical drainage.Drug administration — ACE inhibitors, potassium sparing diuretics (spironolactone), cyclosporin, non selective beta blockers, long-term heparin use (decrease potassium excretion)Packed red blood cells past the expiration dateCrushing injuriesAcute tumour lysis syndromeTranslocation of potassium out of cells associated with heatstroke and reperfusion injury |
Clinical signs include bradycardia, electrocardiogram (ECG) changes and muscle weakness as a result of changes in resting membrane potential of muscle cells (Scalf, 2014). Other signs can include diarrhoea, abdominal pain and even flaccid paralysis. The higher the potassium concentration the more excitable (less negative) and closer to the threshold potential the action potential becomes. The action potential becomes weaker, and if the condition is severe enough depolarisation can be impaired (Figure 1). Cardiac muscles are very sensitive to these effects so hyperkalaemia can be extremely dangerous and lead to fatalities. Potassium levels above 7.5 mEq/litre are considered life threatening (Riordan and Schaer, 2015).
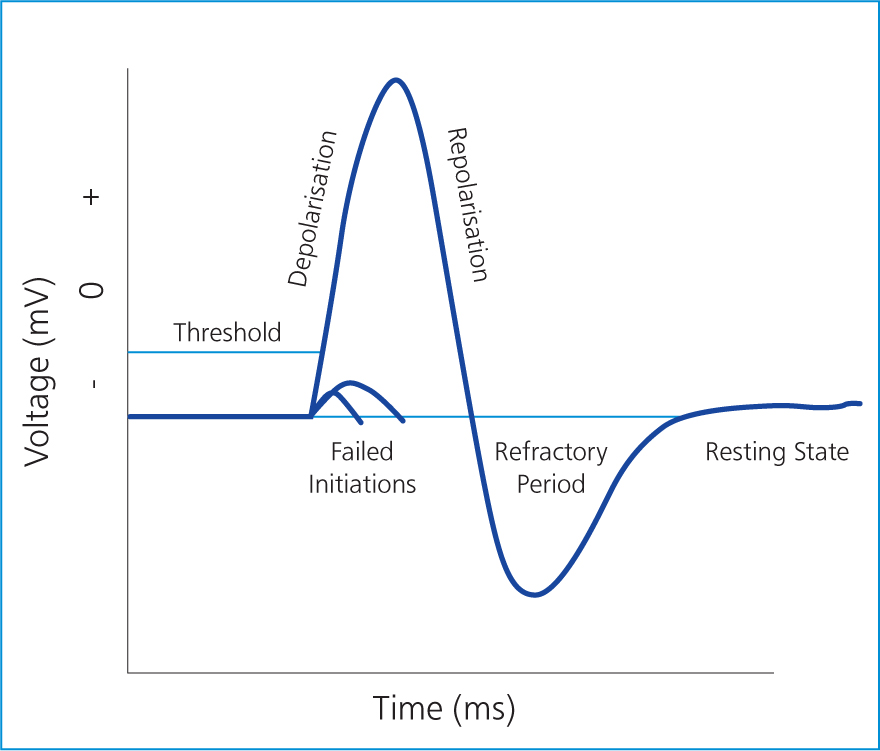
ECG changes vary for each patient as the rate of increase also plays a role, but a general guide is:
- Mild hyperkalaemia 6–7 mEq/litre — high/peaked T waves (Figure 2) (only reported in around 40% of cases) (Scalf, 2014)
- Moderate hyperkalaemia 7–9 mEq/litre — prolonged PR interval and flattened p-waves
- Severe hyperkalaemia 9–10 mEq/litre — atrial standstill (absent p waves) shortening and widening QRS complex (Figure 3)
- >10 mEq/litre — ventricular arrhythmias, fibrillation, sine wave and asystole.
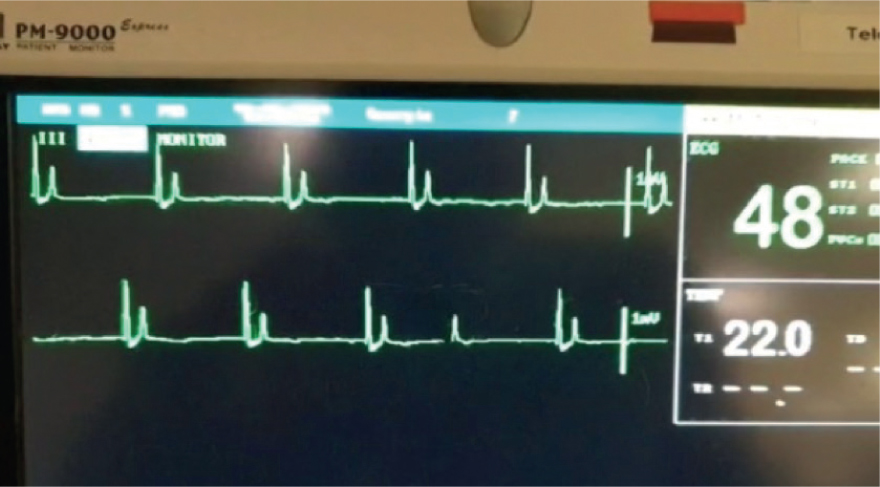
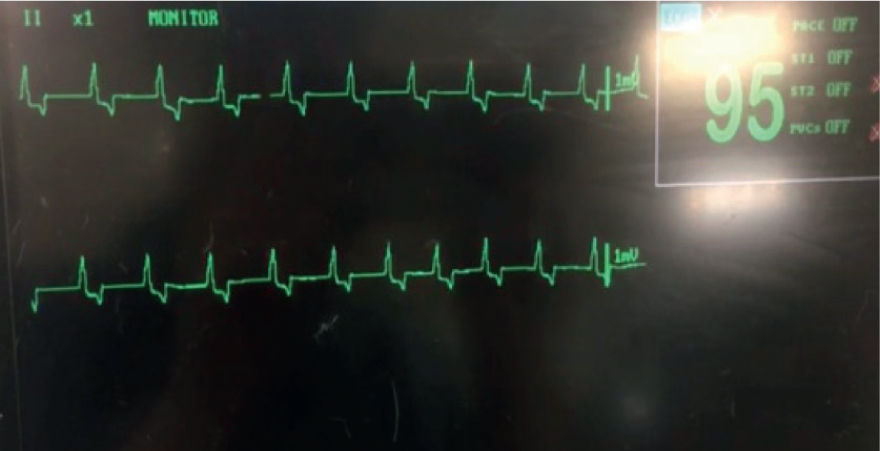
Treatment of hyperkalaemia
The key is to treat the underlying cause while protecting cardiac cells from the effects of hyperkalaemia. Intravenous fluids can be used in cases with normal renal function to promote diuresis. If cardiac signs are apparent then insulin, dextrose and calcium gluconate can be used. Insulin works by promoting potassium cellular influx — soluble insulin is recommended in a dose range of 0.5–1 iu/kg intravenously (Scalf, 2014).
Dextrose is used to counteract hypoglycaemia induced by insulin administration, but also promotes endogenous insulin secretion, thereby decreasing extracellular potassium levels. Dextrose should be diluted before administration and any constant rate infusions above 5% concentrations should be administered via a central line/long-stay catheter because of the hyperosmolar nature of the fluid increasing risk of phlebitis and tissue necrosis if perivascular leakage occurs.
Calcium gluconate 10% solution can be administered at 0.5–1.5 ml/kg intravenously, slowly after dilution (Riordan and Schaer, 2015). Onset of action is around 5 minutes and close ECG monitoring for bradycardia and cardiac arrhythmias is required during administration. Calcium gluconate increases the voltage threshold of an action potential allowing cells to have normal excitability and therefore protecting the heart from the negative effects of potassium, it does not decrease potassium serum levels and effects only last for around 1 hour (Brainard, 2011; Svalf, 2014).
Sodium bicarbonate can be used to increase (alkalise) extracellular pH. Potassium moves into cells to replace hydrogen ions moving extracellularly in response. Therapy works within 1 hour and effects last several hours. Care should be taken in renal compromised patients and those at risk of hypoventilation because of an increase in carbon dioxide produced in sodium bicarbonate conversion. Negative acid–base effects can also be seen. Doses of 1–2 mEq/litre are delivered intravenously over 10–15 minutes (Riordan and Schaer, 2015).
Terbutaline and salbutamol are both beta-adrenergic agonists that increase translocation of potassium into the cell by stimulating the Na/K ATPase pump. Intramuscular or intravenous terbutaline can be given in a dose of 0.01 mg/kg (Riordan and Schaer, 2015), and salbutamol is administered via an inhaler. Tachycardia can occur as a side effect.
Hypokalaemia
The definiton of hypokalaemia is a serum potassium concentration below 3.5 mEq/litre (Riordan et al, 2015) (Table 5).
Table 5. Causes of hypokalaemia
Decreased dietary intakeIncreased losses through gastrointestinal or renal systemsIntracellular translocation (i.e. through alkalosis)Insulin administrationIncreased levels of catecholaminesBeta adrenergic agonist drugs or intoxicationRefeeding syndromeDiabetic ketoacidosisRenal diseaseHyperaldosternonismSevere diarrhoeaChronic liver diseasePost obstructive diuresisDiuretics |
Clinical signs of hypokalaemia
The most significant abnormality as a result of hypokalaemia is skeletal muscle weakness because of decreased excitability of myocyte plasma membranes. A stiff stilted gait, plantigrade stance and ventroflexion of the neck can be noted (Bartges, 2008; Schaer, 2008). Cats are more susceptible to neck ventroflexion because of the absence of a nuchal ligament that in dogs attaches from the axis to the thoracic vertebrae and supports the neck.
Rhabdomyolysis has been noted to occur at potassium levels below 2 mEq/litre (Scalf, 2014).
In myocardial cells a high ratio intracellular/extracellular potassium concentration induces a state of electrical hyperpolarisation leading to prolongation of the action potential predisposing them to atrial and ventricular tachyarrhythmias or even fibrillation (Riordan and Schaer, 2015). Hyperpolarisation increases excitability in cardiac myocytes, particularly the Purkinje fibres, and enhances pacemaker (‘funny’) currents and faster phase 4 depolarisation. (Figure 4).
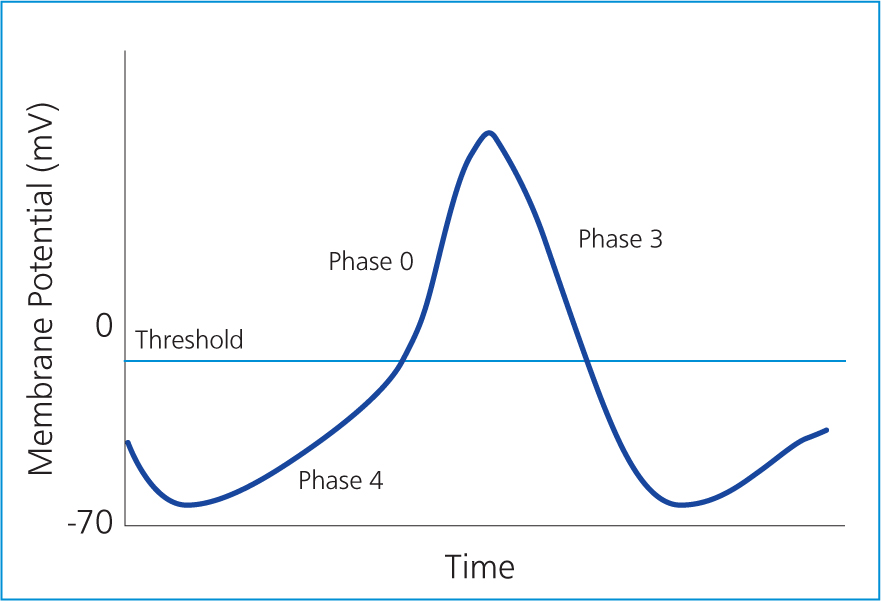
Hypokalaemia causes the myocardium to become refractory to the effects of lidocaine and other class 1 antiarrhythmics (Scalf, 2014).
Treatment of hypokalaemia
Treatment of hypokalaemia involves supplementing with potassium either orally (mild cases) or intravenously (Figure 5). Intravenous infusions should not exceed 0.5 mEq/kg/hour for mild to moderate hypokalaemia. The rate can be cautiously increased to 1 mEq/kg/hour if urine output is normal and if hypokalaemia is profound. High concentrations of potassium supplementation should not be given as a bolus and ECG monitoring is required if the rate exceeds 0.5 mEq/kg/hour.
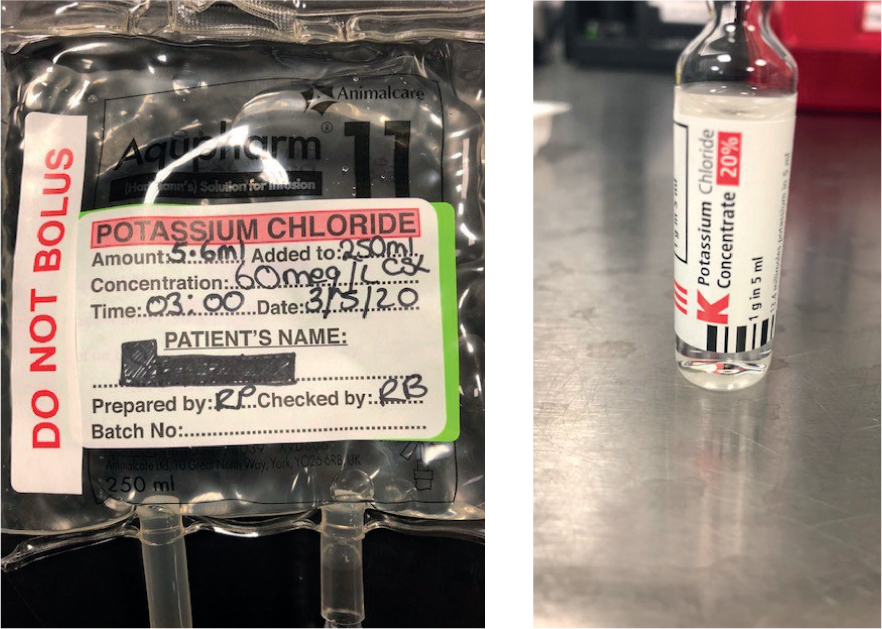
Hypokalaemia that is refractory to supplementation may indicate magnesium levels are low and it has been shown that administration of magnesium in these cases, decreases potassium excretion via the renal outer medullary K+ channel (ROMK). It has been suggested that patients with hypomagnesaemia have a higher prevalence of concurrent hypokalaemia because of altered function of the Na/K ATPase pump (Whang et al, 1984, 1992; Scalf, 2014; Huang and Kuo, 2007). When treating diabetic ketoacidosis it is important to correct potassium to >3.5 mEq/litre before administration of insulin, as failure to do so may lead to life-threatening hypokalaemia secondary to translocation of potassium (Riordan and Schaer, 2015).
Conclusion
It is important to understand the implications of electrolyte derangements especially in the critically ill patient. These patients require monitoring throughout treatment and it is a nurse's role to detect patient abnormalities to prevent impact on fluid balance and physiological processes, delaying recovery and worsening outcome.
KEY POINTS
- It is important to maintain electro neutrality in the body to prevent fluid shifts and adverse side effects.
- By understanding how much each major electrolyte effects the body, it leads to a greater understanding on how to monitor and treat patients presenting with electrolyte abnormalities.
- Potassium is the body's major intracellular cation and disturbances can result in cardiac and musculoskeletal abnormalities which may become life threatening if left untreated.
- Sodium is the body's major extracellular cation and is important in maintaining osmolality and fluid balance.
- The kidneys play a major role in absorption and excretion of electrolytes and water and alongside the gastrointestinal tract and the endocrine system maintain electrolyte balance.