An electrolyte is a substance that dissociates into ions in solution and is capable of transporting an electric charge.
Electrolytes can be positively or negatively charged and are balanced within the body. Positively charged ions are cations and negatively charged are anions.
The major electrolytes in the body include:
- Sodium (Na)
- Chloride (Cl)
- Potassium (K)
- Calcium (Ca)
- Phosphate (PO4)
- Magnesium (Mg).
Electrolytes help regulate fluid balance, nerve and muscle function and maintain blood pH. Concentrations of electrolytes are closely controlled by the action of different hormones and the kidneys. Derangements can affect many body functions including cardiac, skeletal muscle function and the nervous system. The second part of this two part series will look at calcium, phosphate and magnesium.
Calcium
Calcium is crucial for skeletal bone support and numerous intra- and extracellular functions. It is involved in blood coagulation, excitation of neurons, secretion of hormones, enzyme activity and muscle contraction. Ionised calcium mediates acetylcholine release during neuromuscular transmission and stabilises the nerve cell membrane by decreasing its permeability to sodium. 99% of calcium is in bone, the remaining 1% is located in body fluid (Scalf, 2014).
Three forms of circulating calcium exist:
- Ionised (free) — biologically active and considered the most important — 50%
- Protein bound — 40%
- Complexed (bound to bicarbonate, phosphate, citrate and oxalate) — 10%.
Total calcium measures all three components, whereas ionised calcium (Ca2+) measures just the component which is regulated by homeostatic functions. Regulation is complex and involves vitamin D metabolites, calcitonin and parathyroid hormone, all to maintain levels within a narrow margin. Homeostatic functions occur in the gastrointestinal tract, kidney and bone.
Dogs and cats do not effectively synthesise vitamin D in their skin, so need a dietary intake (Green and Chew, 2015).
Acid–base balance, protein levels and chelating agents all affect calcium distribution. Acidosis results in more calcium being ionised and alkalosis results in more being protein bound.
Normal values:
- Total calcium 9–11 mg/dl (young patients may be higher)
- Ionised cat 1.1–1.4 mmol/litre; dog 1.25–1.5 mmol/litre (Green and Chew, 2015).
Hypercalcaemia
Hypercalcaemia is classified by ionised calcium levels >1.4 mmol/litre (Table 1).
Table 1. Causes of hypercalcaemia
Neoplasia |
Vitamin D toxicosis |
Acute kidney injury |
Hypervitaminosis A or D |
Idiopathic |
Hyperparathyroidism |
Calcinosis cutis |
Toxic plants |
Skeletal lesions |
Excessive intake |
Iatrogenic |
Raisin/grape toxicity |
Cholecalciferol (rodenticide) |
Hypoadrenocorticism |
Clinical signs for hypercalcaemia
Clinical signs for hypercalcaemia include lethargy, polyuria, polydipsia, anorexia, vomiting, weakness, constipation, hypertension, twitching, seizures, calcium urolithiasis, cardiac arrhythmias and even death. Raised calcium concentrations depress neurotransmission and has positive inotropic (contractility) and negative chronotropic (rate) effects on the heart leading to bradycardia, prolonged PR interval, widened QRS, wide T waves and may progress to total heart block, asystole and cardiac arrest (Green and Chew, 2015). Calcification of the cardiac muscle and mineralisation of the kidney can occur resulting in irreversible damage.
In cases of neoplasia or paraneoplastic syndrome, hypercalcaemia is caused by osteolytic bone metastasis releasing excessive calcium, release of humoral peptides structurally similar to parathyroid hormone-related proteins, dysregulation of enzyme pathways involved in calcium homeostasis and release of cytokines (Nelson, 2002). It is mainly seen in lymphoma, multiple myeloma, anal sac adenoma or squamous cell carcinoma.
Treatment of hypercalcaemia
Intervention is based on magnitude of hypercalcaemia, clinical signs, rate of development and whether there is presence of organ dysfunction. Initial treatment (alongside trying to identify and remove any underlying cause), is administration of sodium chloride 0.9% fluid therapy which is not only calcium free, but the additional sodium ions compete with calcium in renal tubular reabsorption. Fluid therapy dilutes calcium levels and in combination with diuretics promotes excretion. Corticosteroids (dexamethasone 0.1–0.2 mg/kg) inhibit bone resorption (Green and Chew, 2015), decreases intestinal absorption and increases renal excretion of calcium; however it can interfere with diagnosis of lymphoma so should be avoided until diagnostic tests can be performed.
Calcitonin-salmon can be administered subcutaneously in doses of 4–6 iu/kg (Green and Chew, 2015) to reduce formation of osteoclasts. Biphosphonates, like pamidronate, decrease osteoclastic activity and bone resorption, but can take 1–3 days to reach maximum effect. A constant rate infusion diluted in sodium chloride should be administered over 2–4 hours. Oral preparations can cause oesophageal irritation, nausea and abdominal discomfort (Green and Chew, 2015).
Calcium channel blockers can be used if the patient is at risk of death and sodium bicarbonate can be administered to increase body pH resulting in more calcium binding with protein. Urine output should be monitored in all cases and the patient considered for peritoneal or haemodialysis if oliguria/anuria develops.
Hypocalcaemia
Hypocalcaemia is defined as lower than the normal reference range of blood serum calcium. It is more common than hypercalcaemia and the most common cause is hypoalbuminaemia and renal dysfunction (Table 2).
Table 2. Causes of hypocalcaemia
Hypoalbuminaemia |
Chronic or acute renal failure |
Eclampsia |
Pancreatitis |
Rhabdomyolysis |
Hypoparathyroidism |
Iatrogenic due to bilateral thyroidectomy |
Ethylene glycol toxicity |
Blood transfusions (citrate anticoagulants) |
Sepsis/systemic inflammatory response syndrome (SIRS) (negative prognostic indicator in these cases) |
Chelating agents |
Phosphate enemas, intravenous phosphate, bicarbonate, bisphosphonates, diuretic administration |
Acute tumour lysis syndrome |
Protein losing enteropathy/intestinal malabsorption |
Hypovitaminosis D |
Clinical signs of hypocalcaemia
Clinical signs of hypocalcaemia depend on its duration and severity, but lead to increased neuromuscular activity as a result of increases in cell membrane excitability.
Signs include facial rubbing/paw chewing, hyperexcitability, tremors, restlessness, seizures, panting, hyperthermia, vomiting, diarrhoea, anorexia and tetany. If left untreated severe hypocalcaemia can lead to myocardial failure and respiratory arrest.
Treatment of hypocalcaemia
Treatment of hypocalcaemia is based on ionised calcium readings — if the patient has normal ionised, but low total calcium levels then treatment is not required.
Initially treatment involves administration of calcium gluconate (Figure 1) or calcium chloride via slow intravenous infusion. Calcium salts can cause local reactions leading to tissue necrosis and abscess formation if given by other routes. Calcium gluconate is preferred to calcium chloride because it is less irritating if leaked peri-vascularly. The dose for calcium gluconate 10% solution is 0.5–1.5 ml/kg diluted and can take 30–60 minutes for clinical signs to resolve (Green and Chew, 2015). Heart rate and rhythm should be monitored closely and treatment ceased if any bradyarrhythmias occur. The aim of treatment is to not completely normalise calcium, because overcompensation has deleterious effects especially in primary hypoparathy-roidism, where the lack of parathyroid hormone can lead to calciuria even if calcium is within normal limits (Green and Chew, 2015).
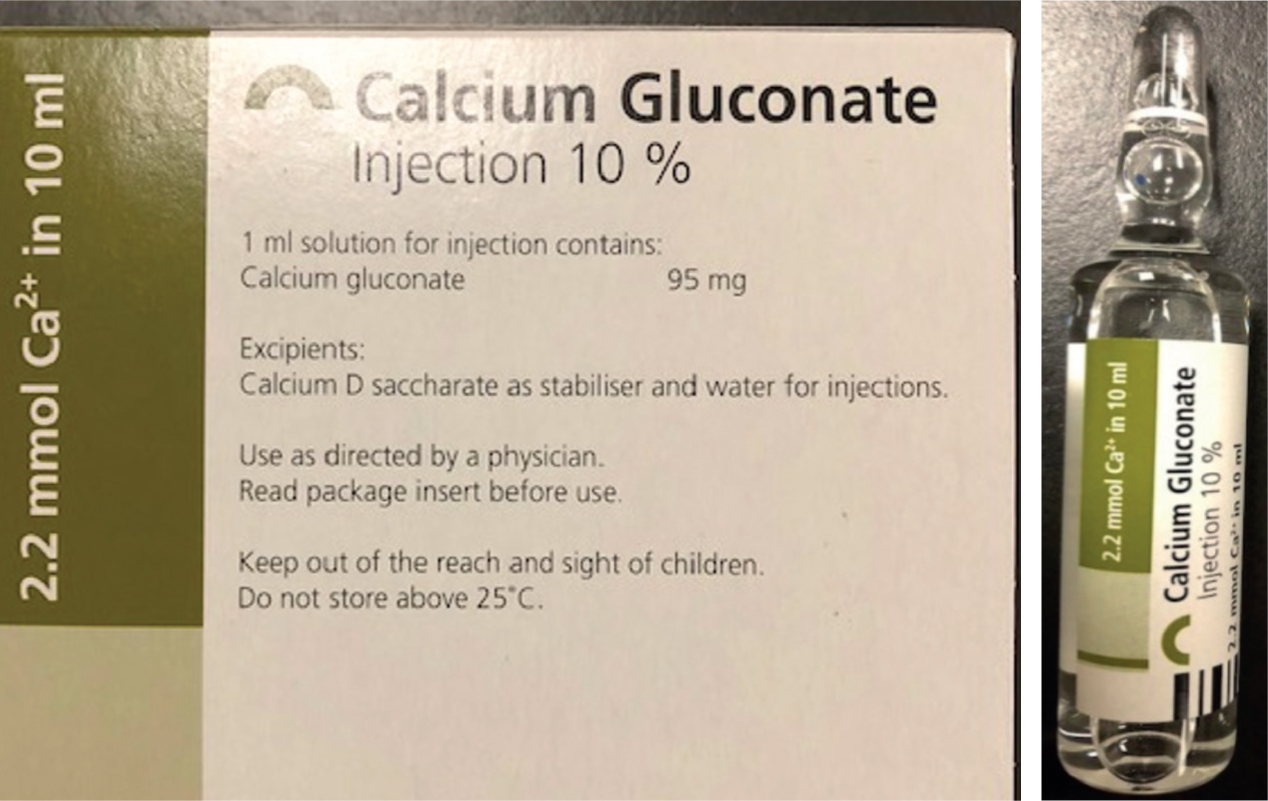
Vitamin D metabolites, if required, should be started as soon as possible because they can take several days for intestinal calcium to be maximised. Calcitriol is usually preferred because it has the quickest onset of action and the shortest half-life. Oral calcium supplements may also be required in cases of hypoparathyroidism or bilateral thyroidectomy.
Phosphate
Phosphorous is essential for biological processes and forms the major intracellular anion phosphate. Phosphate is required for the production of adenosine triphosphate (ATP) so is vital for energy production, metabolic processes such as protein, fat, carbohydrate and nucleic acid metabolism. It maintains cellular membrane integrity and is involved in cell signalling, bone mineralisation and tissue oxygenation by production of 2,3 diphosphoglycerate (DPG) in erythrocytes, which alters the affinity of oxygen to haemoglobin. In addition, it regulates various enzyme activities, buffers acidotic conditions in the body and its major role is maintaining normal bone and teeth matrix (Martin and Allen-Durrance, 2015).
80–90% of total body phosphorous is in bone and teeth, with around 15% is in soft tissue and <1% in extracellular fluid (Scalf 2014).
Serum phosphate is balanced by glomerular filtrate and tubular reabsorption (mainly the proximal convoluted tubule). 80–90% phosphate filtered is reabsorbed. Regulation is through diet, renal excretion, regulatory hormones and movement of phosphorus in and out of cells. The skeleton is used as the body's main store.
Normal levels:
- Cats — 3.0–6.6 mg/dl
- Dogs — 2.5–6 mg/dl (young dogs up to 8.5 mg/dl) (Drobatz and Ward, 2005).
Hypophosphataemia
Hyperphosphataemia Hyperphosphataemia is defined as serum phosphate levels above species and age reference values (Table 3).
Table 3. Causes of hyperphosphataemia
Renal excretion: |
Urethral obstruction |
Uroabdomen |
Hypoparathyroidism |
Hyperthyroidism |
Chronic renal failure (when glomerular filtration rate is 20% below normal) |
Acute renal failure-due to lack of time for compensatory mechanisms |
Increased intake: |
Vitamin D, phosphate enema, iatrogenic |
Transcellular shifts: |
Tumour cell lysis |
Tissue trauma |
Rhabdomyolysis |
Thromboembolic disease |
Haemolysis |
Clinical signs of hyperphosphataemia
The clinical signs of hyperphosphataemia depend on the underlying condition and how rapid the hyperphosphataemia occurred. It can result in seizures, tetany and arrhythmias associated with the development of hypocalcaemia as a result of compensatory mechanisms. Other symptoms include anorexia, nausea, vomiting and soft tissue mineralisation (Martin and Allen-Durrance, 2015).
Treatment of hyperphosphataemia
Identification and treatment of the underlying cause of hyperphosphataemia is required alongside correction of any azotaemia in cases with acute or chronic renal failure. Intravenous fluid therapy with sodium chloride 0.9% will dilute extracellular fluid and enhance renal secretion (Scalf, 2014). Glucose with or without insulin therapy can temporarily reduce serum phosphate levels. Phosphate binders like aluminium hydroxide can be utilised to decrease phosphate absorption in the gastrointestinal tract. A low phosphate diet can also be useful (Scalf, 2014).
In acute kidney injury cases that develop hyperphosphataemia alongside oliguria/anuria, haemodialysis could be considered.
Hypophosphataemia
Hypophosphataemia is characterised by a serum phosphorous level <2.5 mg/dl with clinical signs appearing around 1.5 mg/dl (Scalf, 2014).
Hypophosphataemia is the most common and critical electrolyte disturbance in refeeding syndrome and is caused by depletion of phosphorus levels during starvation followed by release of insulin once re-feeding occurs (Table 4). Insulin promotes intracellular uptake of phosphate and glucose for glycolysis (energy production) and aids tissue damage repair (Drobatz and Ward, 2005; Scalf, 2014).
Table 4. Causes of hypophosphataemia
Decreased intestinal absorption |
Increased urinary excretion |
Transcellular shifts (most common): |
Alkalaemia |
Hyperventilation (respiratory alkalosis) |
Refeeding syndrome |
Parenteral nutrition |
Insulin |
Glucose |
Catecholamines |
Salicylate (Aspirin) toxicity |
Malabsorption: Vitamin D deficiency, phosphate binders/antacids, chronic diarrhoea, steatorrhoea |
Aluminium hydroxide therapy |
Hypothermia |
Clinical signs of hypophosphataemia
Underlying disease, rate and severity all have effects on clinical signs of hypophosphataemia. High energy body cells like erythrocytes, skeletal muscle and brain cells are mainly affected. Neuromuscular signs include weakness, anorexia, ileus, nausea, metabolic encephalopathy, pain as a result of rhabdomyolysis, seizures, stupor or even death. If muscle weakness becomes profound it can affect ventilation, and decreased production of 2,3 DPG increases the affinity of haemoglobin to oxygen, impairing oxygen delivery to tissues leading to hypoxia.
Haemolysis is the most common complication as a result of depleted ATP levels in erythrocytes, making them fragile, less flexible and prone to lysis. Severe hypophosphataemia may impair leukocyte function making patients more susceptible to infections. Platelets have been noted to be affected.
Treatment of hypophosphataemia
Most cases of hypophosphataemia do not require treatment if the underlying cause is successfully treated. In mild cases oral supplementation can be used and are available as sodium, potassium and calcium phosphate salts.
Severe cases require parenteral supplementation in the form of potassium or sodium phosphate solutions. Solutions require dilution before administration and calcium containing fluids should be avoided to prevent precipitation of insoluble calcium phosphate salts.
Doses of 0.01–0.03 mmol/kg of intravenous phosphate (doses up to 0.06 mmol/kg may be required in refeeding syndrome)(Drobatz and Ward, 2005) should be administered intravenously over 6 hours (Figure 2). Hypotension may develop if given rapidly (Scalf, 2014).
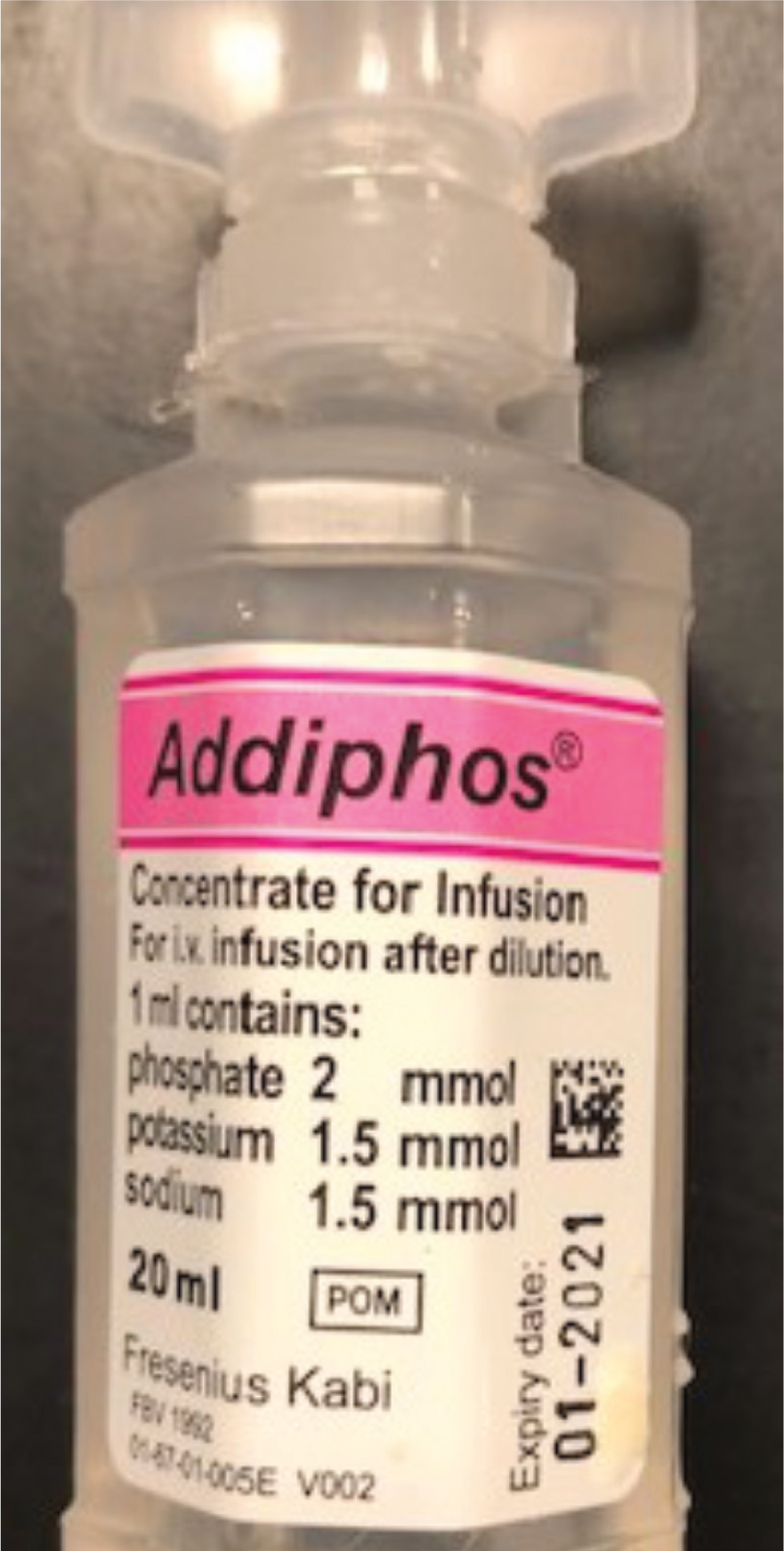
Magnesium
Magnesium is the second most abundant intracellular cation which is mainly found in bone and muscle. It plays a vital role in many organ systems and is a cofactor for ATP production, making it a fundamental electrolyte involved in Na/K ATPase, Ca2+ ATPase and proton pumps (Scalf, 2014). Magnesium is essential for enzyme, protein, nucleic acid synthesis, vascular smooth muscle tone, protein, fat and carbohydrate metabolism, lymphocyte activation, cytokine production and cellular messenger systems. Homeostasis is regulated through intestinal absorption (mainly the jejunum and ileum) and renal excretion. The kidney filters magnesium through glomerular filtrate and the main site of reabsorption is via active transport in the loop of Henle (70%) and passively in the proximal convoluted tubule (30%) (Scalf, 2014; Martin and Allen-Durrance, 2015).
Only 1% of magnesium is present in serum and exists in three states:
- Ionised (physiologically active component)
- Anion complexed
- Protein bound.
Normal reference ranges:
- Ionised 1.07–1.46 mg/dl (0.43–0.7 mmol/l)
- Total canine — 1.6–2.5 mg/dl
- Total feline — 1.9–2.6 mg/dl.
Hypermagnesaemia
Hypermagnesaemia is a less common derangement than hypomagnesaemia because large quantities of magnesium can be excreted by the kidneys. It is unlikely to occur in patients with normal renal function. Hypermagnesemia is defined as a serum magnesium above the normal reference range (> 2.5 mg/dl) (Table 5).
Table 5. Causes of hypermagnesemia
Acute/chronic renal failure |
Cathartics that contain magnesium |
Endocrinopathies |
Iatrogenic |
Clinical signs of hypermagnesaemia
Lethargy, depression and weakness can all occur in patients with hypermagnesaemia, with hyporeflexia occurring because of varying degrees of neuromuscular blockade (Martin and Allen-Durrance, 2015). If the hypermagnesaemia is severe, impaired release of acetylcholine results in respiratory depression, hypoventilation and hypoxaemia (Scalf, 2014).
Treatment of hypermagnesaemia
Treatment for hypermagnesaemia involves diuresis with saline and diuretics, which should be initiated alongside ceasing any medication/supplementation containing magnesium. If the patient has renal impairment then peritoneal or haemodialysis may be considered. Anticholinesterases may be required to offset any neurotoxic effects and calcium administration may antagonise effects on the cardiac muscle (Scalf, 2014). Patients exhibiting signs of respiratory depression may require ventilation. Shock secondary to hypermagnesemia may be refractory to vasopressors, which can make treatment and resuscitation attempts difficult (Scalf, 2014).
Hypomagnesaemia
Hypomagnesaemia is characterised by a serum magnesium level below the reference range (Table 6).
Table 6. Causes of hypomagnesemia
Malnutrition |
Diarrhoea |
Malabsorption — diets containing large amounts of fibre, free fatty acids, oxalate, phosphates or high levels of calcium prevent magnesium absorption due to binding |
Diabetes mellitus |
Hyperthyroidism |
Hyperadrenocorticism |
Hypercalcaemia |
Hypokalaemia |
Loop diuretics |
Renal/tubular disease — drugs that can cause renal tubular damage include aminoglycosides, cyclosporine, cisplastin |
Post obstructive diuresis |
Osmotic agents — hyperglycaemia, mannitol |
Insulin therapy |
Pancreatic insufficiency/pancreatitis |
Sepsis/shock |
Catecholamine excess |
Trauma/burns |
Lactation |
Extended intravenous fluid therapy |
Hypothermia |
Clinical signs of hypomagnesaemia
Clinical signs of hypomagnesaemia mainly consist of neuromuscular (muscle tetany, tremors, seizures, ataxia) and cardiac abnormalities (atrial fibrillation, ventricular tachycardia, supraventricular tachycardia), but can also include anorexia, nausea and ileus (Scalf, 2014).
Being a cofactor for ATP production hypomagnesaemia can result in hypokalaemia and hypocalcaemia predisposing the cardiac muscle to arrhythmias (Bateman, 2006; Scalf, 2014). Magnesium deficiency increases acetylcholine release from nerve and muscle membranes resulting in the neuromuscular signs seen (Martin and Allen-Durrance, 2015).
Treatment for hypomagnesaemia
Supplementation should be considered when levels are below 1.5 mg/dl (Martin and Allen-Durrance, 2015). A 50% dose should be considered in azotaemic patients because of inhibited excretion.
Intravenous continuous rate infusions of 0.25–1 mEq/kg (diluted in D5W or Nacl 0.9%) can be administered for 1–5 days (Figure 3). Side effects of therapy include hypotension, AV block, bundle branch block, but these are usually associated with bolus dosing. Chelation of calcium can occur when using magnesium sulphate, so magnesium chloride is often preferred.
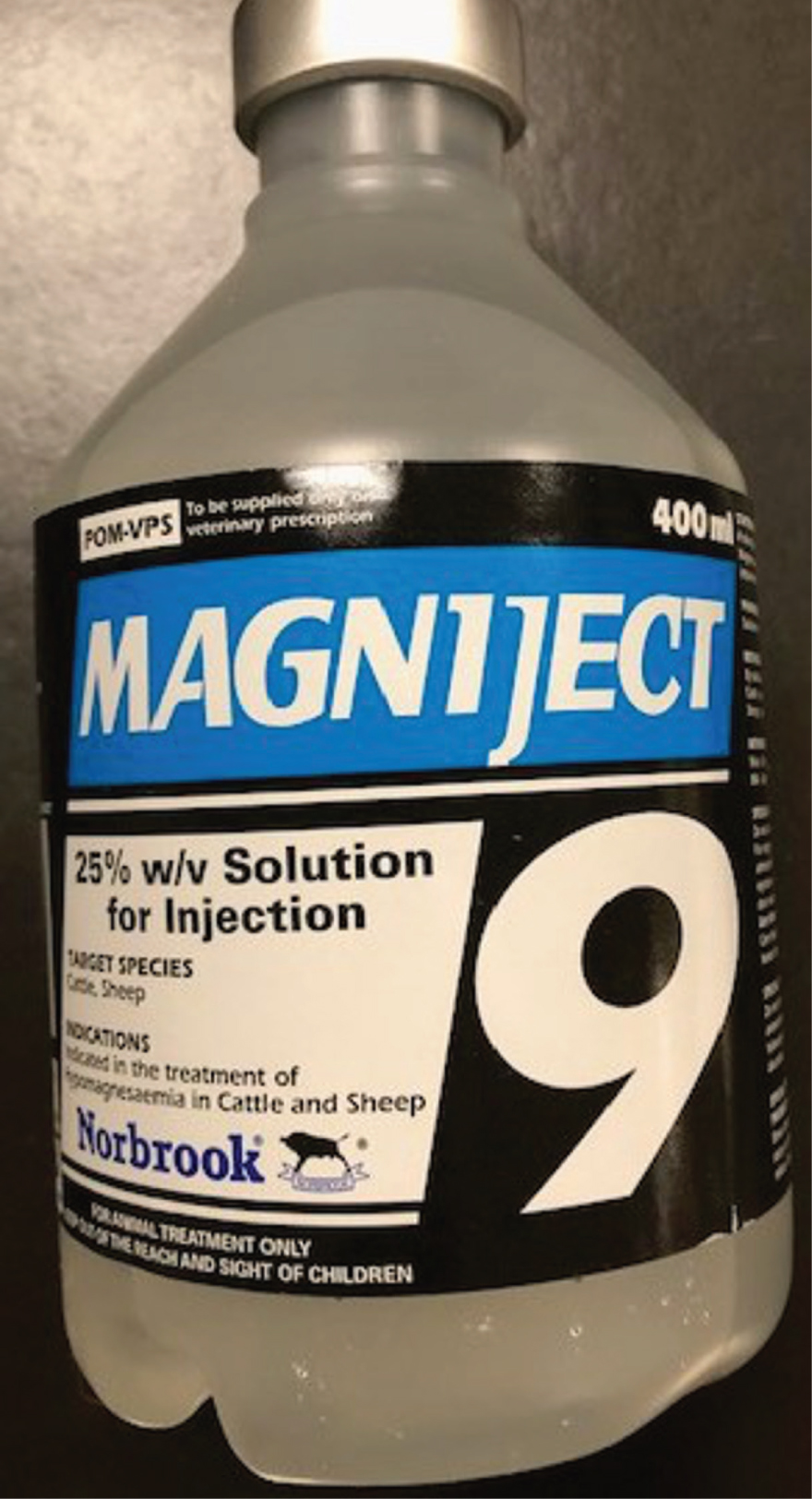
Conclusion
It is important to understand the implications of electrolyte derangements especially in the critically ill patient. These patients require monitoring throughout treatment and it is a nurse's role to detect patient abnormalities to prevent impact on fluid balance and physiological processes, delaying recovery and worsening outcome.
KEY POINTS
- Electrolyte derangements can lead to major body system function disturbances.
- Hypophosphataemia can lead to haemolysis of red blood cells and anaemia.
- Phosphate and calcium disturbances can be interlinked due to compensatory mechanisms.
- The kidneys play an important role in absorption and excretion of all electrolytes.
- Magnesium is a cofactor for ATP production and disturbances result in concurrent abnormalities in other electrolytes including potassium and calcium.
- All three electrolytes mentioned in this part of the series have effects on neuromuscular and cardiac systems when derangements occur.
- Identification of electrolyte abnormalities is important to prevent adverse effects and deterioration in patients.